Anal Fistula Chapter 42 Lower Gastrointestinal Problems-10th Edition-lewis
Abstract
The act of defaecation, although a ubiquitous human experience, requires the coordinated actions of the anorectum and colon, pelvic floor musculature, and the enteric, peripheral and central nervous systems. Defaecation is best appreciated through the description of four phases, which are, temporally and physiologically, reasonably discrete. However, given the complexity of this process, it is unsurprising that disorders of defaecation are both common and problematic; almost everyone will experience constipation at some time in their life and many will develop faecal incontinence. A detailed understanding of the normal physiology of defaecation and continence is critical to inform management of disorders of defaecation. During the past decade, there have been major advances in the investigative tools used to assess colonic and anorectal function. This Review details the current understanding of defaecation and continence. This includes an overview of the relevant anatomy and physiology, a description of the four phases of defaecation, and factors influencing defaecation (demographics, stool frequency/consistency, psychobehavioural factors, posture, circadian rhythm, dietary intake and medications). A summary of the known pathophysiology of defaecation disorders including constipation, faecal incontinence and irritable bowel syndrome is also included, as well as considerations for further research in this field.
Key points
-
Defaecation is a fundamental physiological process resulting in the evacuation of faeces; it is dependent on the coordination of neural, muscular, hormonal and cognitive systems.
-
Several factors influence defaecation, including gastrointestinal transit, stool volume and/or consistency, and dietary intake.
-
Defaecation can be described in terms of four reasonably discrete temporal phases: basal phase, pre-expulsive phase, expulsive phase and end phase.
-
The latest imaging and technological advances (such as high-resolution colonic and anorectal manometry, cine-MRI and magnetic resonance defaecography and wireless capsules) have improved our knowledge of defaecatory mechanisms.
-
Knowledge of the physiology of normal defaecation could inform management of common disorders of defaecation such as constipation and faecal incontinence; however, future research needs are highlighted in this article.
Introduction
Defaecation is a fundamental physiological process that results in the evacuation of faeces. Continence requires the voluntary control of defaecation. Both defaecation and continence are dependent on a morphologically intact gastrointestinal tract and, additionally, the coordination and integration of multiple physiological systems including: neural (principally the enteric nervous system, modulated by the peripheral somatic, autonomic and central nervous systems); muscular (smooth and striated); hormonal (endocrine and paracrine); and cognitive (behavioural and psychosocial)1,2. Disorders of defaecation, such as constipation and faecal incontinence, are common, frequently coexist3,4,5, and incur a considerable burden of morbidity and health-care expenditure6,7,8,9,10. Constipation, for example, is the third most common presenting gastrointestinal symptom reported at outpatient clinics in the USA, with 2.5 million estimated visits in 2014 (ref.11). The direct costs per patient for faecal incontinence and constipation are estimated to be between US$1,594 per year12 and $7,522 per year7.
Since this topic was last reviewed13, there have been major technological advances in the investigative tools used to assess colonic and anorectal function (Box 1) including high-resolution colonic14,15 and anorectal manometry16,17, wireless capsule devices18,19 and MRI techniques20,21,22. In this Review, we provide an overview of the anatomy and physiology of defaecation and continence in human studies, an overview of the pathophysiology of defaecation disorders and summarize considerations for further research (Box 2).
Overview of relevant anatomy
Several structures in the abdomen, pelvis and perineum are integral to defaecation and continence (Fig. 1). We highlight key features in this section, with knowledge gaps and considerations for further research summarized in Box 2.
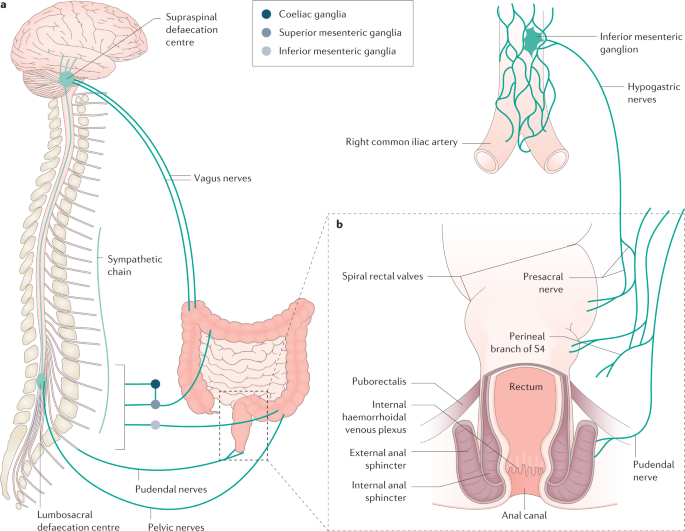
a | Extrinsic sensorimotor innervation of the colon and anorectum relating to the physiology of defaecation. b | A coronal diagram of the anorectum, demonstrating features of structural importance in continence.
Full size image
Colon
The colon is a viscoelastic23, tubular organ, beginning proximally at the ileocaecal junction and ending distally at the rectosigmoid junction. The human colon is approximately 130 cm in length in adulthood24, with a luminal diameter of 60–80 mm in the caecum, progressively narrowing to 25 mm in the sigmoid colon25. An elongated or redundant colon has been suggested to have a causative role in the pathogenesis of constipation26,27. Delayed colonic transit has been demonstrated with colonic elongation in mice28, but it is unclear whether this has any functional relevance in humans.
The colon receives intrinsic neural innervation from the enteric nervous system, extrinsic sympathetic innervation from the lumbar nerves, and extrinsic parasympathetic innervation from the vagus nerve (proximal colon) and pelvic splanchnic nerves, which collectively govern the sensorimotor function of the colon2 (Fig. 1).
Rectum
The rectum can be considered a specialized distal extension of the colon. Located in the pelvis, its high compliance (distensibility in response to filling) is necessary for accommodation of content immediately prior to defaecation (the rectal 'reservoir')29,30. Although a number of landmarks have been used to define the upper border of the rectum, a consensus group suggested in 2019 that the rectum commences at the mesocolic–mesorectal transition, or the 'sigmoid take off'31. This location corresponds to the convergence of the taenia coli into one continuous sheath of longitudinal muscle31.
Anal canal
The lumen of the anal canal is shaped like an hourglass32, with the middle third being the least distensible region33. The length of the anal canal can be described in terms of the anatomical length34 (dentate line to anal verge), surgical length35 (anorectal junction to anal verge) or functional length36 (a high-pressure zone that exceeds the resting intraluminal rectal pressure). The anal canal is generally longer in men than in women (median functional anal canal length: 2.9 cm in women, 3.6 cm in men)16,36,37.
At rest, the anal canal is angulated at approximately 65–108°38,39 to the superior–inferior axis of the rectum, forming the anorectal angle40. The puborectalis muscle forms a U-shaped sling around the anorectal junction, further supporting the anorectal angle via resting tonic activity (postural reflex). The anal canal contains vascular columns41 (columns of Morgagni) and the haemorrhoidal plexus, which are supported by the conjoint longitudinal muscle, or Treitz's muscle42, which is a continuation of the longitudinal muscle of the rectum in the anal canal43. Collectively, these anatomical features contribute up to 10–20% of anal canal resting pressure44.
The internal anal sphincter (IAS) is the continuation of the circular muscle layer of the rectum, forming a ring of smooth muscle that encases the anal canal circumferentially in a spiral orientation45. The IAS is not under voluntary control, receiving autonomic innervation that mediates IAS relaxation via the release of nitric oxide from non-adrenergic, non-cholinergic neurons46,47 (see the section Anorectal sensorimotor activity). This process differs from the conjoint longitudinal muscle, which contracts in response to cholinergic stimulation48. Owing to the spiral orientation of the IAS, contraction causes shortening and narrowing of the anal canal and relaxation causes lengthening45. The resting tone of the IAS can be neural or myogenic in origin49. The resting IAS tone is responsible for the majority (70–85%) of anal canal resting pressure40,44,50,51. The IAS also exhibits phasic contractile activity (termed 'slow waves' and 'ultra-slow waves') that occur at dominant frequencies of 16–18 cycles per minute (cpm) and 1–3 cpm, respectively52,53,54,55; accordingly, the IAS should be considered as a phasically active muscle that generates tone, rather than a tonic muscle as it is conventionally described55.
In contrast to the IAS, the external anal sphincter (EAS) is composed of skeletal muscle under spinal and cortical control56. The EAS makes a small contribution to anal canal resting pressure, but is largely responsible for generating maximal squeeze pressure and the acute voluntary control of continence50. The EAS is further supported by the action of the transverse perineal and bulbospongiosus musculature, to create a so-called purse string closure at the perineal body57.
Pelvic floor musculature and attachments
The pelvic floor includes the striated muscles of levator ani (pubococcygeus, iliococcygeus and puborectalis) and coccygeus34,58,59, as well as the endopelvic fascia and ligamentous attachments, which support the pelvic viscera and provide attachments to the pelvic wall. The principal ligamentous support is afforded by the pubourethral ligament, uterosacral ligament and cardinal ligament60,61. The urogenital hiatus and rectal hiatus allow passage of pelvic viscera to the perineum, including the urethra and vagina anteriorly and rectum posteriorly. The neuromuscular integrity of the IAS, EAS and pelvic floor are critical to continence59, and are most vulnerable to structural traction injury during vaginal delivery or iatrogenic damage secondary to perianal or anorectal surgery62,63,64,65. Connective tissue disorders such as Ehlers–Danlos syndrome hypermobility type can also lead to laxity of pelvic floor ligaments resulting in descending perineum syndrome66.
Four phases of defaecation
The process of defaecation is best appreciated through the description of four phases (Fig. 2), which are temporally and physiologically reasonably discrete13: basal phase, pre-expulsive phase, expulsive phase and end phase. However, some gaps in knowledge remain (Box 2).
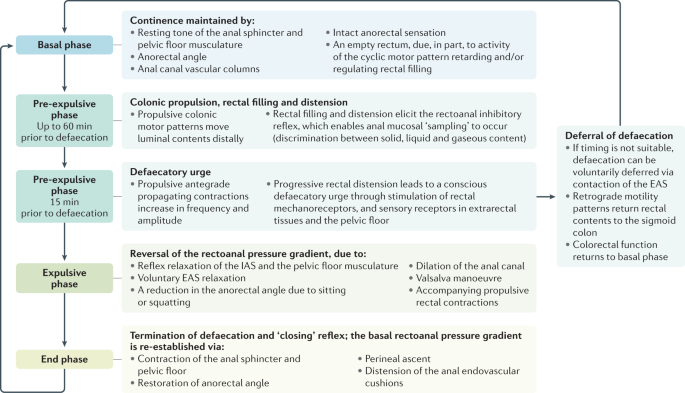
This flowchart depicts the four phases of defaecation (basal, pre-expulsive, expulsive and end), detailing the specific changes occurring during each phase to either maintain continence or facilitate defaecation. EAS, external anal sphincter; IAS, internal anal sphincter.
Full size image
Basal phase
Physiology
The basal phase describes the non-defaecatory state, during which the colon performs several homeostatic functions including67: mixing of luminal content; propulsion of content distally for eventual expulsion; bacterial fermentation of carbohydrates; transmural exchange of fluid, electrolytes and short-chain fatty acids; formation of solid stool; and storage of contents prior to defaecation.
Coordinated motor patterns are essential to these functions68,69. The control of colonic smooth muscle involves the integrated actions of neural, myogenic and hormonal mechanisms1,69, although much of the action, interactions and integration of these systems remains poorly understood70. Continence, simplistically, is maintained by an intraluminal rectoanal pressure gradient, with tonic contraction of the anal sphincter resulting in an anal canal intraluminal resting pressure that exceeds rectal pressure36. The rectum is generally empty during the basal phase and only begins to fill during the pre-expulsive phase59,71.
Colonic motility
The colon receives approximately 1,500 ml of liquid enteric content (chyme) per day via the ileocaecal junction72,73. Mean colonic transit time is ~24 h, ranging between ~4 and 50 h; this represents 70–80% of whole-gut transit time18,19. Digesta enters the caecum and moves aborally (outside episodes of mass movement or defaecation), with a net antegrade flow rate of approximately 1 cm/h (ref.67), and is characterized by a series of 'to-and-fro' motions. As demonstrated by studies tracking an ingestible magnetic capsule, regional transit time in the colon is not evenly distributed74,75 (Supplementary Fig. 1). When the location of the capsule is tracked in real time, it can spend many hours in one region and move through an adjacent region in seconds to minutes75. The motor patterns responsible for these movements might include low-amplitude propagating contractions, high-amplitude propagating contractions (HAPCs), the cyclic motor pattern and colonic pressurizations69.
Low-amplitude propagating contractions and HAPCs, as recorded by intraluminal manometry, have both been temporally associated with luminal transit in humans76,77 and are likely to be the motor patterns associated with the 'fast antegrade' and 'long fast antegrade' movements described in studies using a magnet tracking system18,75. Low-amplitude propagating contractions can also travel in a retrograde direction14,69. These have been associated with retrograde luminal transit and could aid in retarding flow of content through the colon, allowing absorption and fermentation to occur77.
Synchronous pressure increases across long colonic segments, or pancolonic pressurizations, increase during a meal and decrease immediately afterward78,79. These phenomena are associated with transient anal sphincter relaxations78, which enable anal 'sampling' of intraluminal content (discussed below), and also with the flatal urge and expulsion of flatus78,79, which might partly explain the pooling of gas in the distal colon after a meal80. An increase in synchronous colonic pressure waves in the human distal colon was first shown with the administration of the acetylcholinesterase inhibitor neostigmine81, and this finding was confirmed by Corsetti et al. from high-resolution manometry recordings in healthy volunteers78. Similarly, earlier studies using colonic barostat recordings demonstrated increased colonic wall tone in response to meals and neostigmine administration in healthy adults82,83.
Through conventional low-resolution colonic manometry studies, non-propagating activity was commonly reported to be the most prevalent motor pattern in humans84,85. Such activity was suggested to facilitate mixing and transmural exchange of water, electrolytes and short-chain fatty acids. A number of low-resolution manometry studies also identified non-propagating rhythmic activity in the rectum, which was labelled as 'rectal motor complexes'86, 'periodic rectal motor activity'87 or 'intermittent rectal motor activity'88. However, the same motor pattern can be found throughout the entire colon84.
With the introduction of high-resolution manometry, the majority of what was previously considered 'non-propagating' has been shown to consist of rhythmic, propagating contractions, the majority of which occur over short distances at a frequency of 2–8 per minute14. This activity has subsequently been termed the 'cyclic motor pattern', and can be stimulated by a meal14 (discussed below) and HAPCs87,89,90. The cyclic motor pattern is active during sleep86,87 and general anaesthesia91, and can be activated by sacral nerve stimulation in humans92. As it occurs at the same frequency as colonic slow waves93,94,95, the cyclic motor pattern is likely to be generated by the interstitial cells of Cajal94. In addition, the cyclic motor pattern can be modulated by neural pathways in response to physiological, pharmacological and electrical stimulation69,96,97.
Functionally, the cyclic motor pattern in the distal colon has been hypothesized to inhibit transit, thereby acting as an intrinsic colonic 'gatekeeper'87 or 'rectosigmoid brake'15,87,98. Several findings support this hypothesis. For example, the propulsive HAPCs, which are associated with defaecation, increase in number after a meal (see later sections) (Fig. 3). Despite this change, defaecation does not occur after every meal. Concurrently, a meal also results in a major increase in the cyclic motor pattern, which mostly propagates in a retrograde direction. Thus, the presence of the cyclic motor pattern might have a functional role in preventing rectal filling. When an urge to defaecate is perceived, defaecation can be voluntarily deferred, after which time the sensation will usually abate. Radiological evidence suggests that, under such circumstances, retrograde motility patterns can return the contents of the rectum into the sigmoid colon99. This observation is in keeping with findings of an impaired or absent rectosigmoid brake function in patients with conditions characterized by diarrhoea100,101,102,103 and might explain why sacral nerve stimulation can reduce the severity of faecal incontinence by inducing distal colonic motility92,104,105,106.
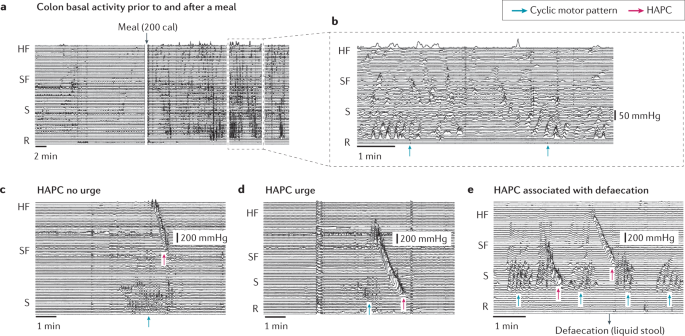
a | The colonic response to a meal. b | Expanded section of the trace outlined (dashed rectangle) in part a showing the cyclic motor pattern (blue arrows). c | The cyclic motor pattern can be seen directly below the high-amplitude propagating contraction (HAPC; magenta arrow); no urge to defaecate was reported by the volunteer. d | By contrast, the HAPC extends into the sigmoid colon (S), the cyclic motor pattern is inhibited and the volunteer reported an urge to defaecate. e | The volunteer reported the passage of a small volume of liquid stool (manometry performed in a prepared colon without solid faecal content). Note that defaecation was associated with a HAPC and inhibition of the cyclic motor pattern. HF, hepatic flexure; R, rectum; SF, splenic flexure.
Full size image
Pre-expulsive phase
Colonic motor activity
Low-resolution colonic manometry in the unprepared colon (with faecal content present) of healthy adults has demonstrated that both propagating and non-propagating activity begins to increase up to 1 h prior to defaecation107. Importantly, these changes are not associated with any conscious awareness or urge. A series of antegrade propagating contractions sequentially originate at a more distal location107. These coordinated motor patterns are likely to move intraluminal content towards the rectum in readiness for evacuation. A study published in 2020 using a magnet tracking system demonstrated distal transit of the capsule from the descending colon to the sigmoid colon 30–60 min prior to defaecation in healthy volunteers (unprepared colon)18.
The functional relevance of increased non-propagating activity prior to defaecation is unknown. As described in the previous section, this activity might assist in slowing antegrade movement. To date, high-resolution colonic manometry studies have only been performed in the prepared colon over short time periods (4–6 h) and, therefore, episodes of spontaneous defaecation are rarely captured108 (Fig. 3).
Anorectal sensorimotor activity
The compliance of the rectal wall allows passive distension, but also adaptive reductions in rectal tone in response to distension, permitting storage of increasing volumes of content with minimal alteration in intraluminal pressure29,109. Rectal distension is detected by mechanoreceptors or rectal intraganglionic laminar endings110, which transmit this information along S2–S4 parasympathetic neurons in the pelvic splanchnic nerves to the spinal cord. There might additionally be mucosal thermoreceptors and chemoreceptors with afferent signalling via spinal nerves; however, this has not been established in animal or human studies111. Sensory receptors are also present in the extrarectal tissues and pelvic floor, as the defaecatory urge can still be perceived in patients following rectal excision with coloanal or ileoanal anastomoses71. Some investigators have suggested that rectal contractions are required to generate a conscious defaecatory urge112,113. During balloon inflation in the rectum, the sensation of rectal distension is not consciously perceived until rectal contractions occur114,115.
Distension of the rectum beyond a threshold initiates the rectoanal inhibitory reflex (RAIR)116, that causes reflex relaxation of the IAS and contraction of the EAS. The RAIR is an intramural reflex mediated by the myenteric plexus and is characteristically absent in Hirschsprung disease, in which the affected segment of rectum and/or colon lacks myenteric ganglia117. Intramural mediation is further evidenced by preservation of the RAIR in patients following spinal cord injury118, or following surgical mobilization and extrinsic denervation of the rectum119. In patients with an enlarged or hypercompliant rectum (for example, megarectum) allied to rectal hyposensitivity30, the RAIR is still present; however, substantially higher rectal volumes are required to deform the rectal wall and elicit the reflex than those required in healthy volunteers120,121.
Transient IAS relaxations occur approximately seven times per hour, and a proportion of these (~40%) might be consciously perceived122. The upper third of the anal canal is the region of greatest compliance33 and, during these transient relaxations, intraluminal pressures within the proximal anal canal equalize with rectal pressures123. This process enables luminal content to be 'sampled' by the mucosa of the anal canal56,122,124, whereby specialized sensory receptors are present including Meissner's corpuscles (touch), Golgi–Mazzoni bodies and Pacinian corpuscles (pressure), Krause end-bulbs (thermal) and genital corpuscles (friction)40,125,126. Sampling of content allows sensory discrimination between solid, liquid and/or gas59,127,128.
The sensory information gathered from anal canal sampling is relayed to the lumbosacral defaecation centre in the spinal cord via parasympathetic neurons within the pelvic splanchnic nerves (S2–S4)129. These afferent neurons include both myelinated Aδ fibres and unmyelinated C fibres130. A spinal cord reflex arc can mediate contraction of the EAS56, while sensory information is additionally relayed to the brainstem and cerebral cortex via the spinothalamic tracts131.
Central nervous system
The conscious perception of rectal distension involves multiple cortical areas including the prefrontal cortex, anterior cingulate gyrus, insula, thalamus and somatosensory cortex132,133. The awareness of rectal filling is graded by the extent of distension, from a mild awareness initially to maximum tolerance115. Cortical input is critical to both voluntary inhibition or initiation of defaecation; notably, patients with spinal cord injury who lack cortical input require stimulation via manual digitation to initiate defaecation134. Brainstem motor control of colonic, rectal and IAS smooth muscle is located in the pontine micturition centre (Barrington's nucleus)135. Motor efferent neurons have cell bodies in Onuf's nucleus in the ventral sacral spinal cord. These neurons return to the anal canal via the inferior rectal branches of the pudendal nerves136,137, whereby they elicit inhibition and relaxation of the anal sphincter136.
Expulsive phase
Colonic motility
In the 15-min period preceding defaecation, antegrade propagating contractions in the colon increase in both frequency and amplitude107. The site of origin of propagating contractions also migrates during this period, with each subsequent propagating event commencing at a more proximal location107. Unlike the pre-defaecatory phase, these propagating contractions are associated with the urge to defaecate. Studies using a magnet tracking system have shown that the capsule can move from the ascending colon to the rectum during this period75. Stool expulsion can be associated with HAPCs that span the entire length of the colon107,138,139, emptying the colon from caecum to rectum140,141. However, stool expulsion can also occur in the absence of HAPCs107, which might require more voluntary effort via abdominal wall contraction.
Manometry studies78,79 in healthy adults have also shown synchronous pressurizations across the distal pressure channels at the termination of HAPCs. This is hypothesized to function as a means to maintain colonic wall tone and facilitate transit as the lumen distal to a propagating contraction dilates to accommodate propulsion of content142,143. These events are additionally associated with rectal balloon expulsion79.
The cyclic motor pattern is inhibited during stool expulsion, presumably to allow intraluminal content to enter the rectum and anal canal. Although never specifically studied in humans or animals, the cyclic motor pattern seems to be inhibited during HAPCs that either terminate in the rectum or are associated with defaecation (Fig. 3d,e).
Rectoanal pressure gradient
In contrast to the basal and pre-expulsive phases, during which anal canal pressure exceeds rectal pressure, the rectoanal pressure differential is, in theory, reversed during the expulsive phase. This pressure gradient exceeds the frictional resistance of the anal canal144 and provides the necessary yield stress to deform solid faeces to enable transit through the anal canal145. This is facilitated by both voluntary and involuntary processes.
A reduction in anal pressure is elicited via a number of factors including voluntary relaxation of the EAS and a reduction in the acuity of the anorectal angle from 65–108° to 110–155°39,146,147. The acuity of the anorectal angle can be further reduced by squatting, hip flexion and/or posterior pelvic tilt148,149, or via the use of a defaecation postural modification device150 (discussed below). Moreover, reflex relaxation of the IAS and pelvic floor musculature and dilation of the anal canal can reduce anal pressure. The extent of IAS relaxation is graded by the rectal stool volume, in that greater stool bulk will cause greater rectal distension and elicit more marked IAS relaxation and reduction in anal canal pressure151. For pelvic floor musculature and anal canal dilation, this contribution might be a combination of passive dilation to accommodate stool, as well as active dilation elicited by perineal descent and contraction of the conjoint longitudinal muscle145,152. Finally, to reduce pressure, conjoint longitudinal muscle contraction flattens the anal endovascular cushions153 and shortens and widens the anal canal43,154, an action which lead Shafik to describe the conjoint longitudinal muscle as the 'evertor ani'155. Simultaneously, an increase in rectal pressure is produced by performing a Valsalva manoeuvre (contraction of the diaphragm and abdominal wall musculature with a closed glottis to increase intra-abdominopelvic pressure) and there might additionally be low-amplitude, propulsive rectal contractions59,156; however, the contribution of rectal wall contractions to increasing intraluminal pressure during the expulsive phase is unclear71.
Disturbance of the normal reversal of the rectoanal pressure gradient during attempted evacuation is described as 'dyssynergic defaecation', a functional disorder of defaecation characterized by failure of relaxation or paradoxical contraction of the anal canal and/or a failure to increase intrarectal pressure157. Dyssynergic defaecation is generally described after anorectal manometric investigation of patients who report difficult evacuation. However, in several studies, a majority of healthy volunteers have also demonstrated a negative rectoanal pressure gradient (that is, anal pressure exceeding rectal pressure) during simulated defaecation158,159,160. Thus, while a reversal of the rectoanal pressure gradient changes may explain stool expulsion conceptually, the investigative tools by which to optimally investigate this process have not yet been determined.
Anal canal dilation
It has been suggested that reflexive anal canal relaxation alone would be insufficient to permit evacuation of faeces; instead, Petros and Swash proposed an active anorectal opening mechanism during defaecation60,152 (Fig. 4). Using defaecography (MRI and video myography), they demonstrated an increase in anorectal luminal diameter during defaecation. The ratio of rectal to anal luminal diameter decreased from approximately 4:1 at rest to 2:1 during defaecation144. They proposed that this action was elicited by simultaneous muscle action in three directional vectors (anterior, posterior and inferior)152, and was achieved by: straightening of the anorectal angle via relaxation of the puborectalis and contraction of the levator plate (posterior vector) and conjoint longitudinal muscle of the anus (inferior vector)152; and actively increasing the luminal diameter of the anal canal via contraction of the pubococcygeus to shift the perineal body (anterior vector) and contraction of the postanal plate to splint the posterior wall of the anal canal (posterior vector)152.
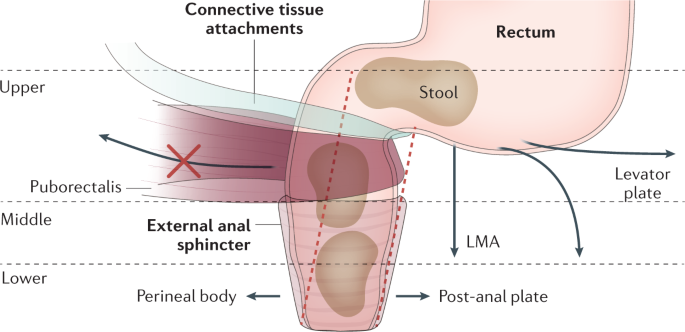
A hypothesis for the anatomical basis of defaecation as detailed by Petros et al.152. Connective tissue attachments anchor the anterior wall of the rectum to the vagina and uterosacral ligaments. Several mechanical changes occur to facilitate defaecation: (1) a reduction in the acuity of the anorectal angle (red dashed lines) is enabled by relaxation of the puborectalis (marked by a red 'X') as well as contraction of the levator plate and conjoint longitudinal muscle of the anus (LMA); (2) the rectoanal pressure gradient is reduced by relaxation of the external anal sphincter; and (3) active dilation of the anal canal occurs via anterior displacement of the perineal body caused by pubococcygeus contraction and posterior movement of the post-anal plate. Adapted from ref.152, Springer Nature Limited.
Full size image
Evacuation
Dynamic assessment of defaecation has been performed using the balloon expulsion test161, 'push' manoeuvres during anorectal manometry162, fluoroscopic or MRI defaecography21, artificial stool163 (Fig. 5), and, more recently, biomechtronics devices such as Fecobionics (a synthetic stool device that provides data on anorectal geometry and manometry during defaecation)164,165. In MRI studies in healthy volunteers, rectosigmoid and total colonic volume was reduced by 44% and 19%, respectively, during defaecation166. Colonic gas volume was also reduced following defaecation in healthy adults, but only in the distal colon167. Preliminary findings in three healthy adults using the Fecobionics device have demonstrated that some expelled the device using a single, sustained pressure effort, whereas others used several abdominopelvic pressure efforts165. Similar patterns of expulsion have been demonstrated in healthy adults using defaecography39. When expelling a slurry of barium sulfate, oats and water, the three patterns observed were: a single, rapid expulsive motion (type 1); frequent, pulsatile expulsion of small volumes (type 2); and slow, sustained, steady expulsion (type 3)39.
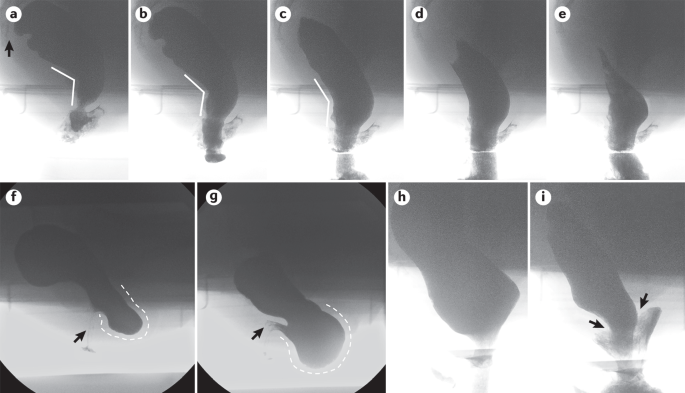
Barium defaecography images provide representative examples, obtained by the senior author from reviewing studies performed in the GI Physiology Unit at Barts Health NHS Trust at the Royal London Hospital, UK (S.M.S. is the director of that clinical service). All images have been anonymized. Barium defaecography provides a real-time anatomical and dynamic study of defaecation, involving fluoroscopic imaging of the process, rate and completeness of rectal emptying, and providing morphological information regarding the anorectum. Parts a–e show defaecation of neostool contrast in a healthy woman (lateral view, the sacrum is indicated by the arrow in part a). There is clear opening of the anorectal angle (parts a–c, angled lines), with progressive dilation of the anal canal (parts b–d) that allows rapid expulsion of most of the contrast. Parts f and g show the development of a huge retaining rectocele (dashed white lines) in a woman complaining of difficulty in rectal evacuation, sense of prolapse and post-defaecation leakage (part f at rest, part g at mid-evacuation). Marked distortion of the anal canal position can be noted (arrows). Parts h and i show the development of a striking full-thickness rectoanal intussusception (arrows) in a man presenting with coexistent faecal incontinence and tenesmus.
Full size image
End phase
This phase represents termination of defaecation and the 'closing reflex'. Following evacuation, a series of changes occur to re-establish the basal rectoanal pressure gradient and restore continence. The closing reflex is theorized to be initiated by cessation of traction on the anal sphincter109. This process elicits: contraction of the anal sphincter and pelvic floor; relaxation of the conjoint longitudinal muscle of the anal canal to enable distension of the anal endovascular cushions; contraction of puborectalis to restore the anorectal angle; and perineal ascent.
Colonic motility patterns in the immediate post-defaecation period have not been described in animal or human studies. In the human stomach and small intestine, prolonged quiescent periods occur after events such as phase III of the migrating motor complex168. However, based on years of colonic manometry studies performed by the authors (C.H.K., P.J.L., P.G.D., S.M.S.; unpublished work), there is no clear period of quiescence following defaecation before motility returns to basal activity. This notion is supported by the consistency of the colonic meal response that occurs irrespective of its temporal proximity to defaecation, and occurs in the prepared colon, demonstrating that it is independent of colonic intraluminal volumes and distension.
Frequency of normal defaecation
Frequency of defaecation varies widely in healthy adults but is most commonly reported as between three bowel motions per day and three bowel motions per week in both sexes169,170,171,172,173,174,175,176,177. The largest study assessing stool frequency in healthy adults included 4,775 participants (2,304 women, 2,471 men) in the USA, of whom 95% self-reported a stool frequency within this range171. These findings are consistent with those from smaller samples in China (1,952 participants)175, the UK (1,897 participants176 and 1,055 participants169), the USA (1,128 participants177 and 789 participants178), Iran (1,045 participants)174, Singapore (271 participants)179, Italy (140 participants)170 and Sweden (124 participants)172.
Compared with adults, the normal frequency of defaecation is substantially higher in infants, particularly during the first month of life. In 240 healthy infants studied in the UK, the mean stool frequency was four bowel motions per day at 2 weeks of age, decreasing to two bowel motions per day by 12 weeks of age180. Mean stool frequency gradually decreases during the first few years of life towards the frequency observed in adults181.
Factors influencing defaecation
Multiple factors influence defaecation, including psychobehavioural factors, diet and sex. Knowledge gaps and considerations for further research for this section are summarized in Box 2.
Psychobehavioural factors and stool withholding
The relationships between cortical activity and gastrointestinal function have been described for over a century182. Stress and psychosocial factors might alter colonic motility183,184,185,186,187 and can contribute to the onset and severity of functional gastrointestinal diseases188 (now also known as 'disorders of gut–brain interactions' (DGBIs)). Contributing factors can include acute and chronic stressors, psychiatric diseases, personality disorders and a history of abuse189. These factors can lead to local alterations in autonomic function, including activation of the hypothalamus–pituitary–adrenal axis and descending modulatory pathways187,190,191, which can influence gastrointestinal motility, visceral afferent signalling190 (hypersensitivity or hyposensitivity), vascular tone and gastrointestinal secretions. Heart rate variability may be used to measure autonomic function; patients with constipation-predominant symptoms show decreased parasympathetic influence (through either withdrawal or sympathetic dominance) compared with patients with diarrhoea-predominant symptoms. The same tendency is seen in patients with severe abdominal pain compared with those with moderate pain, and patients with depression and anxiety compared with those without192. Symptoms can be compounded by hypervigilance, somatization and maladaptive illness behaviours189. Thus, bidirectional brain–gut interactions have a role in the pathogenesis of functional gastrointestinal disorders or DGBIs and psychiatric disorders193,194,195,196. In a prospective longitudinal study, Koloski et al.193 demonstrated that anxiety was predictive of later developing a functional gastrointestinal disorder in 1,002 participants (based on self-reported survey responses at an interval of 12 years and assessed using the Rome II criteria). Conversely, participants with a functional gastrointestinal disorder in the initial survey were found to have higher incidence of anxiety and depression 12 years later193.
Voluntary behaviours also influence defaecation. Toilet training, the process of establishing continence, usually begins between 21 and 36 months of age197 and can take over 7 months to complete198. The voluntary suppression and deferral of defaecation, or stool withholding, is seen most commonly in children and is implicated in the pathophysiology of constipation199. Stool withholding is thought to be associated with painful or unpleasant defaecation and can result in faecal retention, constipation and overflow incontinence3,200,201. In adults, learned behaviours via operant conditioning are also the basis for biofeedback therapy in disorders of defaecation202. Using visual and/or auditory feedback, patients are able to rehearse the activation and coordination of abdominopelvic musculature during defaecation157,203,204.
Posture
Posture has a major influence on the biomechanics of the anorectum205. There are cultural differences in the postures assumed during defaecation, with a squatting position more common in African, Asian and Middle Eastern cultures, and a seated position more common in Western cultures. Squatting increases hip flexion and posterior pelvic tilt, facilitating straightening of the anorectal angle149. When assessed with fluoroscopy, the mean anorectal angle in six healthy volunteers during defaecation in squatting was 126°, compared with 100° in sitting149. Squatting is associated with a reduction in the duration of defaecation, as well as an increased sense of complete evacuation when compared with sitting (mean duration of defaecation: 0.85 min with squatting, 2.16 min with sitting; P < 0.0001)206.
From a seated position, leaning forward to adopt the 'Thinker' position (like the Rodin sculpture Le Penseur) also increases hip flexion148. This position, when compared with sitting upright, was shown on anorectal manometry to increase intrarectal pressure207 and, on cinedefaecography, to result in greater puborectalis relaxation, straightening of the anorectal angle, increased perineal plane distance and improved ease of evacuation of barium neostool148. However, in another study assessing the anorectal angle with fluoroscopy, there was no statistically significant difference in the anorectal angle comparing a seated position and a forward-leaning seated position149.
In a Western population, the use of a foot stool or 'defaecation postural modification device'150 to partially replicate the biomechanics of squatting can result in a reduction in straining and the duration of defaecation and an increase in the perceived completeness of evacuation150. However, these findings were self-reported and unquantified, and have been refuted by others207.
Colonic transit, stool volume and consistency
Faeces is composed predominantly of water (median water content 75%; mean range 63–86% across studies)208, in addition to a suspension of bacterial biomass, protein, carbohydrates and lipids208,209. An analysis combining a distribution of the means from 116 studies in healthy adults found a median faecal wet mass of 128 g per day (mean range 51–796 g per day across studies)208. Stool volume increases considerably in diarrhoeal illnesses, which is predominantly due to an increase in water volume210. Enormous increases in stool water volume, in excess of 10 l per day, can be seen in severe, secretory diarrhoeal illnesses such as cholera211.
Stool consistency is determined by the proportion of solid matter to fluid and is commonly described using the Bristol stool form scale212, which includes a range in consistency from stool type 1 ('separate hard lumps, like nuts') to type 7 ('watery, no solid pieces'). In healthy adults, normal stool consistency has considerable variation, from stool type 2 ('sausage-shaped but lumpy') to type 6 ('fluffy pieces with ragged edges, a mushy stool')171,213. Extremes of stool consistency, from hard stools to watery stools, are associated with slow and rapid colonic transit, respectively214. Lewis and Heaton initially designed the Bristol stool form scale in order to estimate transit time212. Using a combination of radiopaque marker studies and stool diaries, it was demonstrated that whole-gut transit time was most strongly correlated with stool consistency, followed by stool volume and stool frequency212. Jarunvongvanich et al.215 demonstrated similar findings in a Thai population sample, with the Bristol stool form score independently associated with both colonic transit time (faster transit with softer stools) and stool frequency (more frequent stools of softer consistency). Using simultaneous radiopaque markers and wireless motility capsules, Saad et al.216 found that Bristol stool form types 1 and 2 were predictive of delayed whole-gut transit in 46 patients with chronic constipation (sensitivity 85%, specificity 82%). However, in contrast to previous studies, there was no correlation between consistency, transit or stool frequency in the healthy control group (n = 64).
Stool consistency is considerably softer in infancy, with ~60–80% of healthy infants demonstrating soft or liquid stools181,217,218. Stools tend to be firmer in formula-fed infants than in breast-fed infants. In a study including 911 infants (78.2% breast-fed), the prevalence of firm stools was 1.1% in breast-fed infants and 9.2% in formula-fed infants217. Stool consistency seems to normalize from early childhood onwards, with no difference observed in children between the ages of 4 and 15 years219. Similarly to adults, stool consistency is strongly correlated with whole-gut transit time in children219.
Work published in 2020 on the rheology of human faeces demonstrated that stool consistency alters faecal yield stress, which describes the pressure required to deform the faeces to enable rectoanal transit145. Bannister et al.151 used balloons and beads of differing size, volume and consistency to demonstrate that soft, large, deformable balloons were more easily evacuated than harder, smaller beads, requiring a shorter time and lower rectal pressure, and with more complete evacuation in healthy adults.
Colonic transit and stool consistency are interrelated with colonic microbiota composition, diversity and metabolism220,221. Microbial composition is altered by diet and transit time, which can, in turn, alter host physiology222. While causal associations between the gut microbiota and bowel dysfunction remain unclear223, longer colonic transit times can be associated with a shift in colonic metabolism from carbohydrate fermentation to protein catabolism220, reduced short-chain fatty acid concentrations (probably through increased colonic absorption rather than decreased production)224,225, and increased abundance of methanogens221 (as indicated by positive methane breath tests following carbohydrate challenge, which is more common in patients with slow-transit constipation226,227. However, independent of transit time, one study has demonstrated that the colonic microbiota profile has a 94% accuracy for discriminating between healthy adults and patients with constipation (25 women in each group)228. Further studies have demonstrated that the Prevotella-predominant enterotype is associated with softer stool consistency than the Ruminococcus–Bacteroides-predominant enterotype221. Despite these findings, a systematic review published in 2019 demonstrated no differences in colonic microbiota when comparing patients with diarrhoea-predominant and constipation-predominant irritable bowel syndrome (IBS)229. Therapeutic modulation of the colonic microbiome using prebiotics, probiotics, synbiotics and antibiotics for the treatment of gastrointestinal diseases is of great interest230,231,232,233,234,235,236,237,238; however, the specific mechanisms relating colonic microbiota and colonic function are yet to be determined.
Circadian rhythm
Colonic motility exhibits diurnal variation and, in humans, can be inhibited by sleep and increased following morning waking84,85,239,240,241. Propulsive HAPCs can be associated with morning waking and also with the morning call to defaecate107.
Colonic motor response to a meal
Over 100 years ago, eating a meal was identified as a stimulus for 'mass movements' of colonic content242. This colonic response was termed the 'gastrocolonic reflex'243. More recently, the colonic meal response was hypothesized to be a neurohormonal response to gastric distension in humans, causing the release of neuropeptides including cholecystokinin, serotonin, neurotensin and gastrin244. However, the colonic response to a meal can occur independently of gastric stimulation, which was demonstrated by the presence of the colonic meal response following the smell of food or verbal discussion of a meal in healthy adults245, and the preservation of the response in patients after gastrectomy246. Although still commonly used in current journals and textbooks, the term gastrocolonic reflex is, therefore, misleading.
The colonic meal response occurs rapidly. Snape et al. demonstrated an increase in contractility of the sigmoid colon and rectum in 16 healthy adults within minutes of starting to eat a 1,000-calorie meal247. The intensity of the colonic meal response is dependent on the nutritional content of the meal. For example, a meal of 300 calories has a less marked effect on colonic motor function than a meal of 1,000 calories247 and dietary fats cause a greater increase in colonic contraction than carbohydrates248,249. The colonic meal response must be mediated in part by the central nervous system, as this response is absent in patients with spinal injury250 and can be inhibited by the muscarinic receptor antagonist clidinium bromide251.
Low-resolution colonic manometry studies have shown that meals are temporally associated with an increase in non-propagating and low-amplitude propagating contractions throughout the colon84,85,241,252. In addition, HAPCs (which can be associated with defaecation84,85,253) are seen more frequently in the post-prandial period84,85,241. With the introduction of high-resolution colonic manometry (10–30 mm spacing between recording sensors), the timing and characteristics of the colonic meal response have been described in greater detail. Within 60 s of starting a 700-calorie meal, a substantial increase in cyclic motor activity, predominantly propagating in a retrograde direction, occurs in the rectosigmoid region (2.8 events per hour pre-prandial compared with 17.4 events per hour post-prandial; P < 0.001)14,15. Other high-resolution colonic manometry studies have shown that synchronous intraluminal pressure increases (termed pan-colonic pressurizations)78 also increase in frequency during a meal78.
Influence of dietary intake
Dietary intake alters the composition of luminal content, colonic microbiota254 and bowel function255. Dietary fibre, found in cereals, fruits, vegetables and legumes, comprises carbohydrates that are poorly absorbed in the upper gastrointestinal tract. Different fibre sources can be described in terms of their water solubility (water soluble or water insoluble) or their amenability to fermentation by the colonic microbiota (degradable or non-degradable), or categorized by volume of intake as high residue or low residue (high-fibre or low-fibre diets)256.
Degradable fibres are fermented in the colon and increase stool volume predominantly via an increase in bacterial biomass, which can account for over half of the total dry stool volume209,257. Degradable fibres include fermentable oligosaccharides, disaccharides, monosaccharides and polyols (FODMAPs) and resistant starches, and are often synonymous with prebiotics, defined in a consensus statement published in 2017 as compounds within the diet that are selectively utilized by colonic microbiota to confer a health benefit258. A systematic review and meta-analysis demonstrated that an increased dietary intake of resistant starch (22–45 g per day) decreased stool pH, and increased stool volume and stool butyrate concentration, but had no effect on stool frequency259. Using MRI in 14 healthy volunteers, consumption of kiwifruit260 increased retention of water in the small bowel and ascending colon and increased the volume of colonic contents, whereas a high-FODMAP diet261 was associated with an increase in small bowel luminal water content and colonic gas volume in 16 healthy volunteers.
Fibre sources less amenable to colonic fermentation, such as cereal fibres (for example, wheat fibre or psyllium husk), confer a greater increase in stool volume than fermentable fibres262,263. In a study investigating the relationship between diet and colonic content using MRI, non-gaseous colonic content was substantially higher in participants on a high-residue diet (616 ml) than in those on a low-residue diet (479 ml; P = 0.038)167. A systematic review and weighted regression analysis including 65 studies demonstrated that, for every 1 g increase in wheat fibre, stool volume increased by 3.7 ± 0.09 g per day264. Wheat fibre consumption was associated with an increase in stool water content, stool frequency and an apparent normalization of delayed whole-gut transit time in healthy adults262,264. A vegetarian diet, which in most cases contains a high-fibre content, was associated with a statistically significant increase in stool frequency in healthy adults when compared with healthy adults on an omnivorous diet; however, the difference was minimal (11.8 ± 4.5 bowel motions per week on a vegetarian diet compared with 11.3 ± 4.7 bowel motions per week on an omnivorous diet; P < 0.05)173.
Age, sex, parity and BMI
Defaecation is influenced by age, sex and BMI. Most published studies265,266,267,268,269 have shown that ageing is associated with a higher prevalence of constipation (17% prevalence in those aged ≥ 60 years267). However, a 2020 population-based survey of nearly 6,000 adults in the USA, Canada and the UK found a significantly higher prevalence of constipation in those aged 18–34 years (9.9%) than in those aged >65 years (6.5%)270. This finding is in contrast to earlier North American epidemiological studies that demonstrated a higher prevalence of constipation in those aged > 60 years (23.3%) than in the overall population (12.8%)268,269. Both scintigraphic271,272 and wireless ingestible electromagnetic capsule transit studies18 demonstrate longer colonic and whole-gut transit time with increasing age (0.26% increase in colonic transit time per year of life; P = 0.021)18. An age-related decrease in cholinergic function in the ascending colon has also been demonstrated273. However, the cause of altered bowel function in older people is difficult to pinpoint, as a multitude of other changes occur with ageing that could contribute, including diet, medications and decreased physical activity273,274.
In children, the prevalence of constipation is equally distributed between the sexes but, in adults, constipation is reported more commonly by women267,275. Prolonged colonic transit times have been demonstrated in radiopaque marker studies276,277,278,279, scintigraphic studies272,280 and wireless capsule studies18,19 in healthy women. Women also report less frequent bowel motions179 and have greater variability in stool consistency than men (Bristol stool form types 3–5 in men, types 2–6 in women)171, with softer stool consistency during the perimenstrual period281 and firmer stools during the postpartum period281. Variability in bowel habit between the sexes has been hypothesized to be due to cyclical fluctuations in sex hormones282. In one study, exogenous progesterone administered to postmenopausal women accelerated colonic transit (colonic geometric centre at 48 h: 3.2 in women receiving placebo, 3.9 in women receiving progesterone) and resulted in softer stool consistency283. However, other studies have demonstrated slower transit during the luteal phase of the menstrual cycle, during which serum progesterone levels reach their peak214,284,285, or no variation in stool consistency or colonic transit during the luteal phase and follicular phase214.
In terms of anorectal function, men have a greater functional anal canal length16,36,37 and higher rectal sensory thresholds to mechanical distension than women286,287. Women are at risk of pelvic floor and anal sphincter injury during pregnancy and childbirth. Anal sphincter injury is reported in approximately one-third of primiparous women288,289,290, yet less than one-third of women with sphincter injuries report faecal incontinence during the postpartum period291. In some women, the onset of symptoms can be delayed, often occurring decades after pregnancy292,293,294.
Parity can be associated with a reduction in anal canal squeeze pressure16. In women with faecal incontinence or constipation, each successive child is associated with a mean reduction in anal canal resting tone of 4.3 cmH2O and prolongation of pudendal nerve terminal motor latencies295. However, there is only a weak correlation between anal sphincter resting and squeeze pressures and faecal incontinence symptom severity296,297. In the absence of direct anal sphincter injury, a Swedish observational population-based study published in 2019 including over 3.7 million women demonstrated that both caesarean section and vaginal delivery were associated with a risk of developing faecal incontinence, suggesting that other pregnancy-related factors are also involved in the pathogenesis of faecal incontinence (185,219 women with caesarean section only, 1,400,935 with vaginal delivery only)293. Vaginal delivery is also a risk factor for developing descending perineum syndrome, which can be associated with evacuation disorders and chronic constipation66.
The effects of BMI on colonic and anorectal function have been assessed using scintigraphy298, wireless electromagnetic ingestible capsules18 and anorectal investigations37. In a study including 72 participants, there was a trend towards more rapid colonic transit on scintigraphy in patients with a BMI of >30 kg/m2, but the difference was not statistically significant after adjusting for sex298. When assessed with wireless capsules, a higher BMI was significantly related to shorter whole-gut transit time (P = 0.012)18. In a Swedish study including 1,001 people, obesity was additionally associated with stool urgency and the sensation of incomplete rectal evacuation299. Obesity is also an independent risk factor for faecal incontinence300. Among 96 healthy women, an increase in BMI was correlated with a longer balloon expulsion time (reflecting impaired evacuatory efficiency) and a higher threshold volume during rectal sensory testing37.
Other influences
A multitude of other factors influence the physiology of defaecation, some of which include comorbidities, many common medications and physical activity (Box 3). It is beyond the scope of this review to describe each in detail.
Many common medications alter bowel function (Box 3). Opioids, for example, are the most frequently prescribed drug class in the USA301 and are associated with constipation in >40% of patients with chronic non-cancer pain302,303. It is well acknowledged that opioids delay gut transit, but opioid use is also associated with rectal hyposensitivity and 'functional' evacuation disorders in patients with constipation304.
A direct relationship between exercise and bowel function is not clear173. Decreased stool frequency and new onset of constipation was shown in six of ten healthy volunteers after a strict 35-day period of bed rest305. Conversely, 'runner's diarrhoea' can be induced by periods of high-intensity running training (1–2 h per day) in endurance athletes, resulting in higher stool frequency, softer stool consistency, and more rapid small bowel and distal colonic transit than periods of inactivity306.
Common disorders of defaecation
Parameters that constitute a disorder of defaecation are ill-defined. They include the obvious, where defaecation is difficult to initiate or complete (constipation) or control (incontinence), but also include related syndromes such as functional diarrhoea and IBS. Some sense of where diagnoses start and end is provided by the Rome IV criteria using specific combinations of symptoms to define syndromes307,308. For constipation, for example, the Rome IV criteria allow categorization of disorders into four subtypes: functional constipation, IBS with constipation (IBS-C), opioid-induced constipation and functional defaecation disorders. However, there is considerable overlap between these groups and accumulating clinical and mechanistic evidence suggests that these subtypes actually exist on a spectrum rather than as distinct entities309,310,311. It is further acknowledged that it is sometimes difficult to distinguish one from another, and that transition from one functional bowel disorder or from one predominant symptom to another is common. Specifically, considerable overlap between IBS-C and functional constipation exists with regard to both symptoms (for example, abdominal pain and presence of hard stools)311 and pathophysiology (for example, visceral sensory dysfunction, functional evacuation disorders and transit abnormalities)310,311,312,313,314, and transition from functional constipation to IBS-C, and vice versa, is common311,315. Likewise, as noted previously, constipation and faecal incontinence are not distinct conditions, with >40% of patients having concurrent symptoms of both disorders3,4,5,316,317. Regrettably, pathophysiological findings do not neatly equate with syndromes. Rather, it is possible to categorize common abnormalities affecting each of the four phases of defaecation and then note where these have been documented for various clinical syndromes (Table 1).
Full size table
Conclusions
Our understanding of the physiology of defaecation and continence (and also the pathophysiology of conditions such as constipation and faecal incontinence) has progressed considerably, although fundamental uncertainties still remain, particularly regarding the actions, interactions and integration of the myogenic, neural and hormonal mechanisms involved in colonic and anorectal function. It is through resolution of these uncertainties that more effective assessment and individualized treatment of disorders of defaecation will hopefully be achieved. Specific gaps in our knowledge have been summarized in this Review (Box 2), which the authors propose will assist in guiding ongoing research.
References
- 1.
Huizinga, J. D. & Lammers, W. J. Gut peristalsis is governed by a multitude of cooperating mechanisms. Am. J. Physiol. Gastrointest. Liver Physiol. 296, G1–G8 (2009).
CAS PubMed Article Google Scholar
- 2.
Brookes, S. J., Dinning, P. G. & Gladman, M. A. Neuroanatomy and physiology of colorectal function and defaecation: from basic science to human clinical studies. Neurogastroenterol. Motil. 21 (Suppl. 2), 9–19 (2009).
PubMed Article Google Scholar
- 3.
Nurko, S. & Scott, S. M. Coexistence of constipation and incontinence in children and adults. Best Pract. Res. Clin. Gastroenterol. 25, 29–41 (2011).
CAS PubMed PubMed Central Article Google Scholar
- 4.
Cauley, C. E. et al. A quality-of-life comparison of two fecal incontinence phenotypes: isolated fecal incontinence versus concurrent fecal incontinence with constipation. Dis. Colon. Rectum 62, 63–70 (2019).
PubMed Article Google Scholar
- 5.
Vollebregt, P., Wiklendt, L., Dinning, P. G., Knowles, C. H. & Scott, S. M. Coexistent faecal incontinence and constipation: a cross-sectional study of 4,027 adults undergoing specialist assessment. EClinicalMedicine 27, 100572 (2020).
PubMed PubMed Central Article Google Scholar
- 6.
Sun, S. et al. Impact of chronic constipation on health-related quality of life, work productivity, and healthcare resource use: an analysis of the National Health and Wellness Survey. Dig. Dis. Sci. 56, 63–70 (2011).
Google Scholar
- 7.
Nellesen, D., Yee, K., Chawla, A., Lewis, B. E. & Carson, R. T. A systematic review of the economic and humanistic burden of illness in irritable bowel syndrome and chronic constipation. J. Manag. Care Pharm. 19, 755–764 (2013).
PubMed Article Google Scholar
- 8.
Sperber, A. et al. Worldwide prevalence and burden of functional gastrointestinal disorders, results of Rome Foundation global study. Gastroenterology 160, 99–114.e3 (2021).
PubMed Article Google Scholar
- 9.
Peery, A. et al. Burden of gastrointestinal disease in the United States: 2012 update. Gastroenterology 143, 1179–1187 (2012).
PubMed Article Google Scholar
- 10.
Miner, P. B. Jr. Economic and personal impact of fecal and urinary incontinence. Gastroenterology 126, S8–S13 (2004).
PubMed Article Google Scholar
- 11.
Peery, A. et al. Burden and cost of gastrointestinal, liver, and pancreatic diseases in the United States: update 2018. Gastroenterology 156, 254–272 (2019).
PubMed Article Google Scholar
- 12.
Xu, X., Menees, S. B., Zochowski, M. K. & Fenner, D. E. Economic cost of fecal incontinence. Dis. Colon. Rectum 55, 586–598 (2012).
PubMed Article Google Scholar
- 13.
Palit, S., Lunniss, P. J. & Scott, S. M. The physiology of human defecation. Dig. Dis. Sci. 57, 1445–1464 (2012).
PubMed Article Google Scholar
- 14.
Dinning, P. G. et al. Quantification of in vivo colonic motor patterns in healthy humans before and after a meal revealed by high-resolution fiber-optic manometry. Neurogastroenterol. Motil. 26, 1443–1457 (2014).
CAS PubMed PubMed Central Article Google Scholar
- 15.
Lin, A. Y. et al. High-resolution anatomic correlation of cyclic motor patterns in the human colon: evidence of a rectosigmoid brake. Am. J. Physiol. Gastrointest. Liver Physiol 312, G508–G515 (2017).
PubMed PubMed Central Article Google Scholar
- 16.
Carrington, E. V. et al. Traditional measures of normal anal sphincter function using high-resolution anorectal manometry (HRAM) in 115 healthy volunteers. Neurogastroenterol. Motil. 26, 625–635 (2014).
CAS PubMed Article Google Scholar
- 17.
Carrington, E., Knowles, C. H., Grossi, U. & Scott, S. M. High-resolution anorectal manometry measures are more accurate than conventional measures in detecting anal hypocontractility in women with fecal incontinence. Clin. Gastroenterol. Hepatol. 17, 477–485 (2019).
PubMed Article Google Scholar
- 18.
Nandhra, G. et al. Normative values for region-specific colonic and gastrointestinal transit times in 111 healthy volunteers using the 3D-Transit electromagnet tracking system: influence of age, gender, and body mass index. Neurogastroenterol. Motil. 32, e13734 (2020).
PubMed Article Google Scholar
- 19.
Wang, Y. et al. Regional gastrointestinal transit and pH studied in 215 healthy volunteers using the wireless motility capsule: influence of age, gender, study country and testing protocol. Aliment. Pharmacol. Ther. 42, 761–772 (2015).
CAS PubMed Article Google Scholar
- 20.
Kirchhoff, S. et al. Assessment of colon motility using simultaneous manometric and functional cine-MRI analysis: preliminary results. Abdom. Imaging 36, 24–30 (2011).
PubMed Article Google Scholar
- 21.
Grossi, U. et al. Systematic review with meta-analysis: defecography should be a first-line diagnostic modality in patients with refractory constipation. Aliment. Pharmacol. Ther. 48, 1186–1201 (2018).
PubMed Article Google Scholar
- 22.
Lam, C. et al. Colonic response to laxative ingestion as assessed by MRI differs in constipated irritable bowel syndrome compared to functional constipation. Neurogastroenterol. Motil. 28, 861–870 (2016).
CAS PubMed PubMed Central Article Google Scholar
- 23.
Meiss, R. Mechanical properties of gastrointestinal smooth muscle. In Comprehensive Physiology (ed. Terjung, R.) https://doi.org/10.1002/cphy.cp060108 (2011).
- 24.
Phillips, M., Patel, A., Meredith, P., Will, O. & Brassett, C. Segmental colonic length and mobility. Ann. R. Coll. Surg. Engl. 97, 439–444 (2015).
CAS PubMed PubMed Central Article Google Scholar
- 25.
Bass, L. & Wershil, B. K. in Sleisenger and Fordtran's Gastrointestinal and Liver Disease: Pathophysiology/Diagnosis/Management 10th edn Vol. 2 Ch. 98 (eds Feldman, M., Friedman, L. S. & Brandt, L. J.) 1649–1678 (Elsevier Saunders, 2016).
- 26.
Raahave, D., Christensen, E., Loud, F. B. & Knudsen, L. L. Correlation of bowel symptoms with colonic transit, length, and faecal load in functional faecal retention. Dan. Med. Bull. 56, 83–88 (2009).
PubMed Google Scholar
- 27.
Southwell, B. Colon lengthening slows transit: is this the mechanism underlying redundant colon or slow transit constipation? J. Physiol. 588, 3343 (2010).
CAS PubMed PubMed Central Article Google Scholar
- 28.
Heredia, D., Dickson, E. J., Bayguinov, P. O., Hennig, G. W. & Smith, T. K. Colonic elongation inhibits pellet propulsion and migrating motor complexes in the murine large bowel. J. Physiol. 588, 2919–2934 (2010).
CAS PubMed PubMed Central Article Google Scholar
- 29.
Musial, F. & Crowell, M. D. Rectal adaptation to distension: implications for the determination of perception thresholds. Physiol. Behav. 58, 1145–1148 (1995).
CAS PubMed Article Google Scholar
- 30.
Madoff, R., Orrom, W. J., Rothenberger, D. A. & Goldberg, S. M. Rectal compliance: a critical reappraisal. Int. J. Colorectal Dis. 5, 37–40 (1990).
CAS PubMed Article Google Scholar
- 31.
D'Souza, N. et al. Definition of the rectum: an international, expert-based delphi consensus. Ann. Surg. 270, 955–959 (2019).
PubMed Article Google Scholar
- 32.
Jung, S. A. et al. Closure mechanism of the anal canal in women: assessed by three-dimensional ultrasound imaging. Dis. Colon. Rectum 51, 932–939 (2008).
PubMed Article Google Scholar
- 33.
Luft, F. et al. Functional luminal imaging probe: a new technique for dynamic evaluation of mechanical properties of the anal canal. Tech. Coloproctol. 16, 451–457 (2012).
CAS PubMed PubMed Central Article Google Scholar
- 34.
Lee, J. M. & Kim, N. K. Essential anatomy of the anorectum for colorectal surgeons focused on the gross anatomy and histologic findings. Ann. Coloproctol. 34, 59–71 (2018).
PubMed PubMed Central Article Google Scholar
- 35.
Nivatvongs, S., Stern, H. S. & Fryd, D. S. The length of the anal canal. Dis. Colon. Rectum 24, 600–601 (1981).
CAS PubMed Article Google Scholar
- 36.
Vollebregt, P. et al. Functional anal canal length measurement using high-resolution anorectal manometry to investigate anal sphincter dysfunction in patients with fecal incontinence or constipation. Neurogastroenterol. Motil. 31, e13532 (2019).
PubMed Article CAS Google Scholar
- 37.
Oblizajek, N. et al. Anorectal pressures measured with high‐resolution manometry in healthy people — normal values and asymptomatic pelvic floor dysfunction. Neurogastroenterol. Motil. 31, e13597 (2019).
PubMed PubMed Central Article CAS Google Scholar
- 38.
Kim, A. How to interpret a functional or motility test — defecography. J. Neurogastroenterol. Motil. 17, 416–420 (2011).
PubMed PubMed Central Article Google Scholar
- 39.
Palit, S. et al. Evacuation proctography: a reappraisal of normal variability. Colorectal Dis. 16, 538–546 (2014).
CAS PubMed Article Google Scholar
- 40.
Rao, S. S. Pathophysiology of adult fecal incontinence. Gastroenterology 126, S14–S22 (2004).
PubMed Article Google Scholar
- 41.
Gibbons, C. P., Trowbridge, E. A., Bannister, J. J. & Read, N. W. Role of anal cushions in maintaining continence. Lancet 1, 886–888 (1986).
CAS PubMed Article Google Scholar
- 42.
Treitz, W. Ueber einen neuen Muskel am Duodenum des Menschen, uber elsatische Sehnen, und einige andere anatomische Verhaltnisse. Vierteljahrschrift Praktische Heilkunde 37, 133–144 (1853).
Google Scholar
- 43.
Lunniss, P. & Phillips, R. K. S. Anatomy and function of the anal longitudinal muscle. Br. J. Surg. 79, 882–884 (1992).
CAS PubMed Article Google Scholar
- 44.
Lestar, B., Penninckx, F. & Kerremans, R. The composition of anal basal pressure. An in vivo and in vitro study in man. Int. J. Colorectal Dis. 4, 118–122 (1989).
CAS PubMed Article Google Scholar
- 45.
Lunniss, P. Aspects of fistula-in-ano. Master of Surgery thesis, Univ. London (1993).
- 46.
O'Kelly, T., Brading, A. & Mortensen, N. Nerve mediated relaxation of the human internal anal sphincter: the role of nitric oxide. Gut 34, 689–693 (1993).
PubMed PubMed Central Article Google Scholar
- 47.
Chakder, S. & Rattan, S. Release of nitric oxide by activation of nonadrenergic noncholinergic neurons of internal anal sphincter. Am. J. Physiol. Gastrointest. Liver Physiol. 264, G7–G12 (1993).
CAS Article Google Scholar
- 48.
O'Kelly, T., Brading, A. & Mortensen, N. In vitro response of the human and canal longitudinal muscle layer to cholinergic and adrenergic stimulation: evidence of sphincter specialization. Br. J. Surg. 80, 1337–1341 (1993).
PubMed Article Google Scholar
- 49.
Rattan, S. The internal anal sphincter: regulation of smooth muscle tone and relaxation. Neurogastroent Motil. 17 (Suppl. 1), 50–59 (2005).
Article Google Scholar
- 50.
Frenckner, B. & Euler, C. V. Influence of pudendal block on the function of the anal sphincters. Gut 16, 482–489 (1975).
CAS PubMed PubMed Central Article Google Scholar
- 51.
Schweiger, M. Method for determining individual contributions of voluntary and involuntary anal sphincters to resting tone. Dis. Colon. Rectum 22, 415–416 (1979).
CAS PubMed Article Google Scholar
- 52.
Wankling, W. J., Brown, B. H., Collins, C. D. & Duthie, H. L. Basal electrical activity in the anal canal in man. Gut 9, 457–460 (1968).
CAS PubMed PubMed Central Article Google Scholar
- 53.
Kerremans, R. Electrical activity and motility of the internal anal sphincter: an "in vivo" electrophysiological study in man. Acta Gastroenterol. Belg. 31, 465–482 (1968).
CAS PubMed Google Scholar
- 54.
Orkin, B., Hanson, R. B., Kelly, K. A., Phillips, S. F. & Dent, J. Human anal motility while fasting, after feeding, and during sleep. Gastroenterology 100, 1016–1023 (1991).
CAS PubMed Article Google Scholar
- 55.
Keef, K. & Cobine, C. A. Control of motility in the internal anal sphincter. J. Neurogastroenterol. Motil. 25, 189–204 (2019).
PubMed PubMed Central Article Google Scholar
- 56.
Broens, P. M., Penninckx, F. M. & Ochoa, J. B. Fecal continence revisited: the anal external sphincter continence reflex. Dis. Colon. Rectum 56, 1273–1281 (2013).
PubMed Article Google Scholar
- 57.
Mittal, R. K., Bhargava, V., Sheean, G., Ledgerwood, M. & Sinha, S. Purse-string morphology of external anal sphincter revealed by novel imaging techniques. Am. J. Physiol. Gastrointest. Liver Physiol. 306, G505–G514 (2014).
CAS PubMed PubMed Central Article Google Scholar
- 58.
Grigorescu, B. A. et al. Innervation of the levator ani muscles: description of the nerve branches to the pubococcygeus, iliococcygeus, and puborectalis muscles. Int. Urogynecol J. Pelvic Floor. Dysfunct. 19, 107–116 (2008).
PubMed Article Google Scholar
- 59.
Bharucha, A. E. Pelvic floor: anatomy and function. Neurogastroenterol. Motil. 18, 507–519 (2006).
CAS PubMed Article Google Scholar
- 60.
Petros, P. & Swash, M. The musculo-elastic theory of anorectal function and dysfunction. Pelviperineology 27, 89–93 (2008).
Google Scholar
- 61.
Herschorn, S. Female pelvic floor anatomy: the pelvic floor, supporting structures, and pelvic organs. Rev. Urol. 6 (Suppl. 5), 2–10 (2004).
Google Scholar
- 62.
LaCross, A., Groff, M. & Smaldone, A. Obstetric anal sphincter injury and anal incontinence following vaginal birth: a systematic review and meta-analysis. J. Midwifery Womens Health 60, 37–47 (2015).
PubMed Article Google Scholar
- 63.
Snooks, S. J., Setchell, M., Swash, M. & Henry, M. M. Injury to innervation of pelvic floor sphincter musculature in childbirth. Lancet 2, 546–550 (1984).
CAS PubMed Article Google Scholar
- 64.
Snooks, S. J., Swash, M., Mathers, S. E. & Henry, M. M. Effect of vaginal delivery on the pelvic floor: a 5-year follow-up. Br. J. Surg. 77, 1358–1360 (1990).
CAS PubMed Article Google Scholar
- 65.
Hieda, K. et al. Nerves in the intersphincteric space of the human anal canal with special reference to their continuation to the enteric nerve plexus of the rectum. Clin. Anat. 26, 843–854 (2013).
PubMed Google Scholar
- 66.
Wang, X., Chedid, V., Vijayvargiya, P. & Camilleri, M. Clinical features and associations of descending perineum syndrome in 300 adults with constipation in gastroenterology referral practice. Dig. Dis. Sci. 65, 3688–3695 (2020).
PubMed Article Google Scholar
- 67.
Dinning, P., Costa, M. & Brookes, S. J. H. in Sleisenger and Fordtran's Gastrointestinal and Liver Disease 11th edn Vol. 2 Ch. 100 (eds Feldman, M., Friedman, L. S. & Brandt, L. J.) 1595–1610 (Elsevier, 2021).
- 68.
Scott, S. Manometric techniques for the evaluation of colonic motor activity: current status. Neurogastroenterol. Motil. 15, 483–513 (2003).
CAS PubMed Article Google Scholar
- 69.
Corsetti, M. et al. First translational consensus on terminology and definitions of colonic motility in animals and humans studied by manometric and other techniques. Nat. Rev. Gastroenterol. Hepatol. 16, 559–579 (2019).
PubMed PubMed Central Article Google Scholar
- 70.
Spencer, N., Dinning, P. G., Brookes, S. J. & Costa, M. Insights into the mechanisms underlying colonic motor patterns. J. Physiol. 594, 4099–4116 (2016).
CAS PubMed PubMed Central Article Google Scholar
- 71.
Moonka, R. & Carmichael, J. C. in Fundamentals of Anorectal Surgery (eds Beck, D., Steele, S. & Wexner, S.) 1–21 (Springer, 2018).
- 72.
Dinning, P., Costa, M. & Brookes, S. J. H. in Sleisenger and Fordtran's Gastrointestinal and Liver Disease: Pathophysiology/Diagnosis/Management 10th edn Vol. 2 Ch. 100 (eds Feldman, M., Friedman, L. S. & Brandt, L. J.) 1697–1712 (Elsevier Saunders, 2016).
- 73.
Bassotti, G., Battaglia, E. in Colon, Rectum and Anus: Anatomic, Physiologic and Diagnostic Bases for Disease Management (eds Ratto, C., Parrello, A., Dionisi, L. & Litta, F.) 43–53 (Springer, 2017). [Series eds Ratto, C., Parrello, A. & Litta, F. Coloproctology Vol. 1]
- 74.
Haase, A. M. et al. Pilot study trialling a new ambulatory method for the clinical assessment of regional gastrointestinal transit using multiple electromagnetic capsules. Neurogastroenterol. Motil. 26, 1783–1791 (2014).
CAS PubMed Article Google Scholar
- 75.
Mark, E. et al. Ambulatory assessment of colonic motility using the electromagnetic capsule tracking system: effect of opioids. Neurogastroenterol. Motil. 32, e13753 (2019).
PubMed Google Scholar
- 76.
Cook, I. J., Furukawa, Y., Panagopoulos, V., Collins, P. J. & Dent, J. Relationships between spatial patterns of colonic pressure and individual movements of content. Am. J. Physiol.Gastrointest. Liver Physiol. 278, G329–G341 (2000).
CAS PubMed Article Google Scholar
- 77.
Dinning, P. G., Szczesniak, M. M. & Cook, I. J. Proximal colonic propagating pressure waves sequences and their relationship with movements of content in the proximal human colon. Neurogastroenterol. Motil. 20, 512–520 (2008).
CAS PubMed Article Google Scholar
- 78.
Corsetti, M. et al. Pan-colonic pressurisations associated with relaxation of the anal sphincter in health and disease: a new colonic motor pattern identified using high-resolution manometry. Am. J. Gastroenterol. 112, 479–489 (2017).
PubMed Article Google Scholar
- 79.
Chen, J. H. et al. Characterization of simultaneous pressure waves as biomarkers for colonic motility assessed by high-resolution colonic manometry. Front. Physiol. 9, 1248 (2018).
PubMed PubMed Central Article Google Scholar
- 80.
Perez, F., Accarino, A., Azpiroz, F., Quiroga, S. & Malagelada, J. R. Gas distribution within the human gut: effects of meals. Am. J. Gastroenterol. 102, 842–849 (2007).
PubMed Article Google Scholar
- 81.
Deller, D. & Wangel, A. G. Intestinal motility in man. I. A study combining the use of intraluminal pressure recording and cineradiography. Gastroenterology 48, 45–57 (1965).
CAS PubMed Article Google Scholar
- 82.
Law, N., Bharucha, A. E., Undale, A. S. & Zinsmeister, A. R. Cholinergic stimulation enhances colonic motor activity, transit, and sensation in humans. Am. J. Physiol. Gastrointest. Liver Physiol 281, G1228–G1237 (2001).
CAS PubMed Article Google Scholar
- 83.
Corsetti, M., Gevers, A. M., Caenepeel, P. & Tack, J. The role of tension receptors in colonic mechanosensitivity in humans. Gut 53, 1787–1793 (2004).
CAS PubMed PubMed Central Article Google Scholar
- 84.
Bampton, P., Dinning, P. G., Kennedy, M. L., Lubowski, D. Z. & Cook, I. J. Prolonged multipoint recording of colonic manometry in the unprepared human colon: providing insight into potentially relevant pressure wave parameters. Am. J. Gastroenterol. 96, 1838–1848 (2001).
CAS PubMed Article Google Scholar
- 85.
Rao, S. S., Sadeghi, P., Beaty, J., Kavlock, R. & Ackerson, K. Ambulatory 24-h colonic manometry in healthy humans. Am. J. Physiol. Gastrointest. Liver Physiol 280, G629–G639 (2001).
CAS PubMed Article Google Scholar
- 86.
Kumar, D., Williams, N. S., Waldron, D. & Wingate, D. L. Prolonged manometric recording of anorectal motor activity in ambulant human subjects: evidence of periodic activity. Gut 30, 1007–1011 (1989).
CAS PubMed PubMed Central Article Google Scholar
- 87.
Rao, S. S. & Welcher, K. Periodic rectal motor activity: the intrinsic colonic gatekeeper? Am. J. Gastroenterol. 91, 890–897 (1996).
CAS PubMed Google Scholar
- 88.
Prior, A., Fearn, U. J. & Read, N. W. Intermittent rectal motor activity: a rectal motor complex? Gut 32, 1360–1363 (1991).
CAS PubMed PubMed Central Article Google Scholar
- 89.
Hagger, R., Kumar, D., Benson, M. & Grundy, A. Periodic colonic motor activity identified by 24-h pancolonic ambulatory manometry in humans. Neurogastroenterol. Motil. 14, 271–278 (2002).
CAS PubMed Article Google Scholar
- 90.
Pervez, M., Ratcliffe, E., Parsons, S. P., Chen, J. H. & Huizinga, J. D. The cyclic motor patterns in the human colon. Neurogastroenterol. Motil. 32, e13807 (2020).
PubMed Article Google Scholar
- 91.
Vather, R. et al. Hyperactive motility responses occur in the distal colon following colonic surgery [abstract 124]. Neurogastroenterol. Motil. 28 (Suppl. 1), 41 (2016).
Google Scholar
- 92.
Patton, V., Wiklendt, L., Arkwright, J. W., Lubowski, D. Z. & Dinning, P. G. The effect of sacral nerve stimulation on distal colonic motility in patients with faecal incontinence. Br. J. Surg. 100, 959–968 (2013).
CAS PubMed Article Google Scholar
- 93.
Auli, M. et al. Effects of excitatory and inhibitory neurotransmission on motor patterns of human sigmoid colon in vitro. Br. J. Pharmacol. 155, 1043–1055 (2008).
CAS PubMed PubMed Central Article Google Scholar
- 94.
Rae, M. G., Fleming, N., McGregor, D. B., Sanders, K. M. & Keef, K. D. Control of motility patterns in the human colonic circular muscle layer by pacemaker activity. J. Physiol. 510, 309–320 (1998).
CAS PubMed PubMed Central Article Google Scholar
- 95.
Taylor, I., Darby, C. & Hammond, P. Comparison of rectosigmoid myoelectrical activity in the irritable colon syndrome during relapses and remissions. Gut 19, 923–929 (1978).
CAS PubMed PubMed Central Article Google Scholar
- 96.
Dinning, P. G. et al. Neural mechanisms of peristalsis in the isolated rabbit distal colon: a neuromechanical loop hypothesis. Front. Neurosci. 8, 75 (2014).
PubMed PubMed Central Article Google Scholar
- 97.
Rosli, R. et al. Distinct patterns of myogenic motor activity identified in isolated human distal colon with high‐resolution manometry. Neurogastroenterol. Motil. 8, e13871 (2020).
Google Scholar
- 98.
Lin, A., Dinning, P. G., Milne, T., Bissett, I. P. & O'Grady, G. The "rectosigmoid brake": review of an emerging neuromodulation target for colorectal functional disorders. Clin. Exp. Pharmacol. Physiol. 44, 719–728 (2017).
CAS PubMed Article Google Scholar
- 99.
Ritchie, J. Colonic motor activity and bowel function. Gut 9, 442–456 (1968).
CAS PubMed PubMed Central Article Google Scholar
- 100.
Clemens, C. H., Samsom, M., Van Berge Henegouwen, G. P. & Smout, A. J. Abnormalities of left colonic motility in ambulant nonconstipated patients with irritable bowel syndrome. Dig. Dis. Sci. 48, 74–82 (2003).
CAS PubMed Article Google Scholar
- 101.
Cole, S. J. et al. Distal colonic motor activity in four subgroups of patients with irritable bowel syndrome. Dig. Dis. Sci. 47, 345–355 (2002).
CAS PubMed Article Google Scholar
- 102.
Chey, W. Y., Jin, H. O., Lee, M. H., Sun, S. W. & Lee, K. Y. Colonic motility abnormality in patients with irritable bowel syndrome exhibiting abdominal pain and diarrhea. Am. J. Gastroenterol. 96, 1499–1506 (2001).
CAS PubMed Article Google Scholar
- 103.
Kern, F. Jr, Almy, T. P., Abbot, F. K. & Bogdonoff, M. D. The motility of the distal colon in nonspecific ulcerative colitis. Gastroenterology 19, 492–503 (1951).
PubMed Article Google Scholar
- 104.
Payne, S. C., Furness, J. B. & Stebbing, M. J. Bioelectric neuromodulation for gastrointestinal disorders: effectiveness and mechanisms. Nat. Rev. Gastroenterol. Hepatol. 16, 89–105 (2018).
Article Google Scholar
- 105.
Jarrett, M. et al. Sacral nerve stimulation for fecal incontinence related to obstetric anal sphincter damage. Dis. Colon. Rectum 51, 531–537 (2008).
PubMed Article Google Scholar
- 106.
Melenhorst, J., Koch, S. M., Uludag, O., van Gemert, W. G. & Baeten, C. G. Sacral neuromodulation in patients with faecal incontinence: results of the first 100 permanent implantations. Colorectal Dis. 9, 725–730 (2007).
CAS PubMed Article Google Scholar
- 107.
Bampton, P. A. et al. Spatial and temporal organization of pressure patterns throughout the unprepared colon during spontaneous defecation. Am. J. Gastroenterol. 95, 1027–1035 (2000).
CAS PubMed Article Google Scholar
- 108.
Dinning, P. A new understanding of the physiology and pathophysiology of colonic motility? Neurogastroenterol. Motil. 30, e13395 (2018).
CAS PubMed Article Google Scholar
- 109.
Bajwa, A. & Emmanuel, A. The physiology of continence and evacuation. Best Pract. Res. Clin. Gastroenterol. 23, 477–485 (2009).
PubMed Article Google Scholar
- 110.
Ng, K. S., Brookes, S. J., Montes-Adrian, N. A., Mahns, D. A. & Gladman, M. A. Electrophysiological characterization of human rectal afferents. Am. J. Physiol. Gastrointest. Liver Physiol. 311, G1047–G1055 (2016).
PubMed PubMed Central Article Google Scholar
- 111.
Knowles, C. H. Human studies of anorectal sensory function. Ir. J. Med. Sci. 187, 1143–1147 (2018).
PubMed PubMed Central Article Google Scholar
- 112.
Ihre, T. Studies on anal function in continent and incontinent patients. Scand. J. Gastroenterol. Suppl. 25, 1–64 (1974).
CAS PubMed Google Scholar
- 113.
Read, N., Timms, J. M., Barfield, L. J., Donnelly, T. C. & Bannister, J. J. Impairment of defecation in young women with severe constipation. Gastroenterology 90, 53–60 (1986).
CAS PubMed Article Google Scholar
- 114.
Sun, W., Read, N. W. & Miner, P. B. Relation between rectal sensation and anal function in normal subjects and patients with faecal incontinence. Gut 31, 1056–1061 (1990).
CAS PubMed PubMed Central Article Google Scholar
- 115.
Sun, W. M. et al. Sensory and motor responses to rectal distention vary according to rate and pattern of balloon inflation. Gastroenterology 99, 1008–1015 (1990).
CAS PubMed Article Google Scholar
- 116.
Gowers, W. The automatic action of the sphincter ani. Proc. R. Soc. Lond. 26, 77–84 (1877).
Google Scholar
- 117.
Lawson, J. & Nixon, H. H. Anal canal pressures in the diagnosis of Hirschsprung's disease. J. Pediatric Surg. 2, 544–552 (1967).
CAS Article Google Scholar
- 118.
Frenckner, B. Function of the anal sphincters in spinal man. Gut 16, 638–644 (1975).
CAS PubMed PubMed Central Article Google Scholar
- 119.
Lubowski, D. Z., Nicholls, R. J., Swash, M. & Jordan, M. J. Neural control of internal anal sphincter function. Br. J. Surg. 74, 668–670 (1987).
CAS PubMed Article Google Scholar
- 120.
Remes-Troche, J., De-Ocampo, S., Paulson, J. & Rao, S. S. C. Recto-anal reflexes and sensori-motor response in rectal hyposensitivity. Dis. Colon. Rectum 53, 1047–1054 (2010).
PubMed PubMed Central Article Google Scholar
- 121.
Verduron, A. et al. Megarectum. Dig. Dis. Sci. 33, 1164–1174 (1988).
CAS PubMed Article Google Scholar
- 122.
Miller, R., Bartolo, D. C., Cervero, F. & Mortensen, N. J. Anorectal sampling: a comparison of normal and incontinent patients. Br. J. Surg. 75, 44–47 (1988).
CAS PubMed Article Google Scholar
- 123.
Cheeney, G., Nguyen, M., Valestin, J. & Rao, S. S. Topographic and manometric characterization of the recto-anal inhibitory reflex. Neurogastroenterol. Motil. 24, e147–e154 (2012).
CAS PubMed PubMed Central Article Google Scholar
- 124.
Duthie, H. & Bennett, R. C. The relation of sensation in the anal canal to the functional anal sphincter (a possible factor in anal incontinence). Gut 4, 179–182 (1963).
CAS PubMed PubMed Central Article Google Scholar
- 125.
Rogers, J. Testing for and the role of anal and rectal sensation. Baillieres Clin. Gastroenterol. 6, 179–191 (1992).
CAS PubMed Article Google Scholar
- 126.
Duthie, H. L. & Gairns, F. W. Sensory nerve-endings and sensation in the anal region of man. Br. J. Surg. 47, 585–595 (1960).
CAS PubMed Article Google Scholar
- 127.
Broens, P., Vanbeckevoort, D., Bellon, E. & Penninckx, F. Combined radiologic and manometric study of rectal filling sensation. Dis. Colon. Rectum 45, 1016–1022 (2002).
PubMed Article Google Scholar
- 128.
Duthie, H. L. Dynamics of the rectum and anus. Clin. Gastroenterol. 4, 467–477 (1975).
CAS PubMed Article Google Scholar
- 129.
Furness, J. B. The enteric nervous system and neurogastroenterology. Nat. Rev. Gastroenterol. Hepatol. 9, 286–294 (2012).
CAS PubMed Article Google Scholar
- 130.
Gourcerol, G. et al. How sacral nerve stimulation works in patients with faecal incontinence. Colorectal Dis. 13, E203–E211 (2011).
CAS PubMed Article Google Scholar
- 131.
Craig, A. D. B. A new view of pain as a homeostatic emotion. Trends Neurosci. 26, 303–307 (2003).
CAS PubMed Article Google Scholar
- 132.
Bittorf, B., Ringler, R., Forster, C., Hohenberger, W. & Matzel, K. F. Cerebral representation of the anorectum using functional magnetic resonance imaging. Br. J. Surg. 93, 1251–1257 (2006).
CAS PubMed Article Google Scholar
- 133.
Moisset, X. et al. Anatomical connections between brain areas activated during rectal distension in healthy volunteers: a visceral pain network. Eur. J. Pain 14, 142–148 (2010).
PubMed Article Google Scholar
- 134.
Lynch, A. C., Antony, A., Dobbs, B. R. & Frizelle, F. A. Bowel dysfunction following spinal cord injury. Spinal Cord 39, 193–203 (2001).
CAS PubMed Article Google Scholar
- 135.
Browning, K. N. & Travagli, R. A. Central nervous system control of gastrointestinal motility and secretion and modulation of gastrointestinal functions. Compr. Physiol. 4, 1339–1368 (2014).
PubMed PubMed Central Article Google Scholar
- 136.
Welcome, M. O. Gastrointestinal Physiology: Development, Principles, and Mechanisms of Regulation (Springer, 2018).
- 137.
Callaghan, B., Furness, J. B. & Pustovit, R. V. Neural pathways for colorectal control, relevance to spinal cord injury and treatment: a narrative review. Spinal Cord 56, 199–205 (2018).
PubMed Article Google Scholar
- 138.
Kamm, M. A., van der Sijp, J. R. & Lennard-Jones, J. E. Colorectal and anal motility during defaecation. Lancet 339, 820 (1992).
CAS PubMed Article Google Scholar
- 139.
Bassotti, G. & Gaburri, M. Manometric investigation of high-amplitude propagated contractile activity of the human colon. Am. J. Physiol. Gastrointestinal. Liver Physiol. 255, G660–G664 (1988).
CAS Article Google Scholar
- 140.
Halls, J. Bowel content shift during normal defaecation. Proc. R. Soc. Med. 58, 859–860 (1965).
CAS PubMed PubMed Central Google Scholar
- 141.
Lubowski, D., Meagher, A. P., Smart, R. C. & Butler, S. P. Scintigraphic assessment of colonic function during defecation. Int. J. Colorectal Dis. 10, 91–93 (1995).
CAS PubMed Article Google Scholar
- 142.
Mohd, R. et al. Discriminating movements of liquid and gas in the rabbit colon with impedance manometry. Neurogastroenterol. Motil. 30, e13263 (2017).
Google Scholar
- 143.
Dinning, P. G. et al. Temporal relationships between wall motion, intraluminal pressure, and flow in the isolated rabbit small intestine. Am. J. Physiol. Gastrointestinal. Liver Physiol. 300, G577–G585 (2011).
CAS Article Google Scholar
- 144.
Bush, M., Petros, P., Swash, M., Fernandez, M. & Gunnemann, A. Defecation 2: Internal anorectal resistance is a critical factor in defecatory disorders. Tech. Coloproctol. 16, 445–450 (2012).
CAS PubMed Article Google Scholar
- 145.
de Loubens, C. et al. Rheology of human faeces and pathophysiology of defaecation. Tech. Coloproctol. 24, 323–329 (2020).
PubMed Article Google Scholar
- 146.
Piloni, V., Fioravanti, P., Spazzafumo, L. & Rossi, B. Measurement of the anorectal angle by defecography for the diagnosis of fecal incontinence. Int. J. Colorectal Dis. 14, 131–135 (1999).
CAS PubMed Article Google Scholar
- 147.
Tirumanisetty, P. et al. Normal values for assessment of anal sphincter morphology, anorectal motion, and pelvic organ prolapse with MRI in healthy women. Neurogastroenterol. Motil. 30, e13314 (2018).
CAS PubMed PubMed Central Article Google Scholar
- 148.
Takano, S. & Sands, D. R. Influence of body posture on defecation: a prospective study of "The Thinker" position. Tech. Colproctol. 20, 117–121 (2016).
CAS Article Google Scholar
- 149.
Sakakibara, R. et al. Influence of body position on defecation in humans. Low. Urin. Tract. Symptoms 2, 16–21 (2010).
PubMed Article Google Scholar
- 150.
Modi, R. M. et al. Implementation of a defecation posture modification device: impact on bowel movement patterns in healthy subjects. J. Clin. Gastroenterol. 53, 216–219 (2019).
PubMed PubMed Central Article Google Scholar
- 151.
Bannister, J., Davison, P., Timms, J. M., Gibbons, C. & Read, N. W. Effect of stool size and consistency on defecation. Gut 28, 1246–1250 (1987).
CAS PubMed PubMed Central Article Google Scholar
- 152.
Petros, P. et al. Defecation 1: testing a hypothesis for pelvic striated muscle action to open the anorectum. Tech. Coloproctol. 16, 437–443 (2012).
CAS PubMed Article Google Scholar
- 153.
Barleben, A. & Mills, S. Anorectal anatomy and physiology. Surg. Clin. North Am. 90, 1–15 (2010).
PubMed Article Google Scholar
- 154.
Koda, K. et al. Etiology and management of low anterior resection syndrome based on the normal defecation mechanism. Surg. Today 49, 803–808 (2019).
PubMed Article Google Scholar
- 155.
Shafik, A. A new concept of the anatomy of the anal sphincter mechanism and the physiology of defecation. III. The longitudinal anal muscle: anatomy and role in anal sphincter mechanism. Investig. Urol. 13, 271–277 (1976).
CAS Google Scholar
- 156.
Ito, T. et al. Videomanometry of the pelvic organs: a comparison of the normal lower urinary and gastrointestinal tracts. Int. J. Urol. 13, 29–35 (2006).
PubMed Article Google Scholar
- 157.
Rao, S. S. Dyssynergic defecation and biofeedback therapy. Gastroenterol. Clin. North Am. 37, 569–586, viii (2008).
PubMed PubMed Central Article Google Scholar
- 158.
Ratuapli, S., Bharucha, A. E., Noelting, J., Harvey, D. M. & Zinsmeister, A. R. Phenotypic identification and classification of functional defecatory disorders using high-resolution anorectal manometry. Gastroenterology 144, 314–322 (2013).
PubMed Article Google Scholar
- 159.
Grossi, U. et al. Diagnostic accuracy study of anorectal manometry for diagnosis of dyssynergic defecation. Gut 65, 447–455 (2016).
PubMed Article Google Scholar
- 160.
Noelting, J. et al. Normal values for high-resolution anorectal manometry in healthy women: effects of age and significance of rectoanal gradient. Am. J. Gastroenterol. 107, 1530–1536 (2012).
PubMed PubMed Central Article Google Scholar
- 161.
Caetano, A. C., Santa-Cruz, A. & Rolanda, C. Digital rectal examination and balloon expulsion test in the study of defecatory disorders: are they suitable as screening or excluding tests? Can. J. Gastroenterol. Hepatol. 2016, 8654314 (2016).
PubMed PubMed Central Article Google Scholar
- 162.
Palit, S. et al. Diagnostic disagreement between tests of evacuatory function: a prospective study of 100 constipated patients. Neurogastroenterol. Motil. 28, 1589–1598 (2016).
CAS PubMed Article Google Scholar
- 163.
Pelsang, R. E., Rao, S. S. & Welcher, K. FECOM: a new artificial stool for evaluating defecation. Am. J. Gastroenterol. 94, 183–186 (1999).
CAS PubMed Article Google Scholar
- 164.
Gregersen, H., Krogh, K. & Liao, D. Fecobionics: integrating anorectal function measurements. Clin. Gastroenterol. Hepatol. 16, 981–983 (2018).
PubMed Article Google Scholar
- 165.
Sun, D. et al. Fecobionics: a novel bionics device for studying defecation. Ann. Biomed. Eng. 47, 576–589 (2019).
PubMed Article Google Scholar
- 166.
Nilsson, M. et al. Quantification and variability in colonic volume with a novel magnetic resonance imaging method. Neurogastroenterol. Motil. 27, 1755–1763 (2015).
CAS PubMed Article Google Scholar
- 167.
Bendezu, R. A. et al. Colonic content: effect of diet, meals, and defecation. Neurogastroenterol. Motil. 29, e12930 (2017).
Article Google Scholar
- 168.
Deloose, E., Janssen, P., Depoorte, I. & Tack, J. The migrating motor complex: control mechanisms and its role in health and disease. Nat. Rev. Gastroenterol. Hepatol. 9, 271–285 (2012).
CAS PubMed Article Google Scholar
- 169.
Connell, A. M., Hilton, C., Irvine, G., Lennard-Jones, J. E. & Misiewicz, J. J. Variation of bowel habit in two population samples. Br. Med. J. 2, 1095–1099 (1965).
CAS PubMed PubMed Central Article Google Scholar
- 170.
Bellini, M. et al. Self-perceived normality in defecation habits. Dig. Liver Dis. 38, 103–108 (2006).
CAS PubMed Google Scholar
- 171.
Mitsuhashi, S. et al. Characterizing normal bowel frequency and consistency in a representative sample of adults in the United States (NHANES). Am. J. Gastroenterol. 113, 115–123 (2018).
PubMed Article Google Scholar
- 172.
Walter, S. A., Kjellstrom, L., Nyhlin, H., Talley, N. J. & Agreus, L. Assessment of normal bowel habits in the general adult population: the Popcol study. Scand. J. Gastroenterol. 45, 556–566 (2010).
PubMed Article Google Scholar
- 173.
Panigrahi, M. K., Kar, S. K., Singh, S. P. & Ghoshal, U. C. Defecation frequency and stool form in a coastal eastern Indian population. J. Neurogastroenterol. Motil. 19, 374–380 (2013).
PubMed PubMed Central Article Google Scholar
- 174.
Adibi, P., Behzad, E., Pirzadeh, S. & Mohseni, M. Bowel habit reference values and abnormalities in young Iranian healthy adults. Dig. Dis. Sci. 52, 1810–1813 (2007).
PubMed Article Google Scholar
- 175.
Fang, X., Lu, S. & Pan, G. An epidemiologic study of bowel habit in adult non-patient population in Beijing area. Zhonghua Yi Xue Za Zhi 81, 1287–1290 (2001).
CAS PubMed Google Scholar
- 176.
Heaton, K. W. et al. Defecation frequency and timing, and stool form in the general population: a prospective study. Gut 33, 818–824 (1992).
CAS PubMed PubMed Central Article Google Scholar
- 177.
Sandler, R. & Drossman, D. A. Bowel habits in young adults not seeking health care. Dig. Dis. Sci. 32, 841–845 (1987).
CAS PubMed Article Google Scholar
- 178.
Drossman, D., Sandler, R. S., McKee, D. C. & Lovitz, A. J. Bowel patterns among subjects not seeking health care. Use of a questionnaire to identify a population with bowel dysfunction. Gastroenterology 83, 529–534 (1982).
CAS PubMed Article Google Scholar
- 179.
Chen, L., Ho, K. Y. & Phua, K. H. Normal bowel habits and prevalence of functional bowel disorders in Singaporean adults — findings from a community based study in Bishan. Singap. Med. J. 41, 255–258 (2000).
CAS Google Scholar
- 180.
Weaver, L. T., Ewing, G. & Taylor, L. C. The bowel habit of milk-fed infants. J. Pediatr. Gastroenterol. Nutr. 7, 568–571 (1988).
CAS PubMed Article Google Scholar
- 181.
Steer, C. D., Emond, A. M., Golding, J. & Sandhu, B. The variation in stool patterns from 1 to 42 months: a population-based observational study. Arch. Dis. Child. 94, 231–233 (2009).
CAS PubMed Article Google Scholar
- 182.
Pavlov, I. P. The Work of the Digestive Glands (Griffin, 1902).
- 183.
Almy, T. P. Experimental studies on the irritable colon. Am. J. Med. 10, 60–67 (1951).
CAS PubMed Article Google Scholar
- 184.
Welgan, P., Meshkinpour, H. & Beeler, M. Effect of anger on colon motor and myoelectric activity in irritable bowel syndrome. Gastroenterology 94, 1150–1156 (1988).
CAS PubMed Article Google Scholar
- 185.
Welgan, P., Meshkinpour, H. & Hoehler, F. The effect of stress on colon motor and electrical activity in irritable bowel syndrome. Psychosom. Med. 47, 139–149 (1985).
CAS PubMed Article Google Scholar
- 186.
Rao, S. S., Hatfield, R. A., Suls, J. M. & Chamberlain, M. J. Psychological and physical stress induce differential effects on human colonic motility. Am. J. Gastroenterol. 93, 985–990 (1998).
CAS PubMed Article Google Scholar
- 187.
Kano, M. et al. Altered brain and gut responses to corticotropin-releasing hormone (CRH) in patients with irritable bowel syndrome. Sci. Rep. 29, 12425 (2017).
Article CAS Google Scholar
- 188.
Drossman, D. A. Functional gastrointestinal disorders: history, pathophysiology, clinical features and Rome IV. Gastroenterology 150, 1262–1279 (2016).
Article Google Scholar
- 189.
Drossman, D. in Sleisenger and Fordtran's Gastrointestinal and Liver Disease: Pathophysiology/Diagnosis/Management 10th edn Ch. 22 (eds Feldman, M., Friedman, L. S. & Brandt, L. J.) 349–362 (Elsevier Saunders, 2016).
- 190.
Mayer, E. A. & Tillisch, K. The brain–gut axis in abdominal pain syndromes. Annu. Rev. Med. 62, 381–396 (2011).
CAS PubMed Article Google Scholar
- 191.
Clevers, E., Lutin, E., Cornelis, J. & Van Oudenhove, L. Gastrointestinal symptoms in office workers are predicted by psychological distress and short sleep duration. J. Psychosom. Res. 138, 110230 (2020).
PubMed Article Google Scholar
- 192.
Mazurak, N., Seredyuk, N., Sauer, H., Teufel, M. & Enck, P. Heart rate variability in the irritable bowel syndrome: a review of the literature. Neurogastroenterol. Motil. 24, 206–216 (2012).
CAS PubMed Article Google Scholar
- 193.
Koloski, N. et al. The brain–gut pathway in functional gastrointestinal disorders is bidirectional: a 12-year prospective population-based study. Gut 61, 1284–1290 (2012).
CAS PubMed Article Google Scholar
- 194.
Carabotti, M., Scirocco, A., Maselli, M. A. & Severi, C. The gut – brain axis: interactions between enteric microbiota, central and enteric nervous systems. Ann. Gastroenterol. 28, 203–209 (2015).
PubMed PubMed Central Google Scholar
- 195.
Powell, N., Walker, M. M. & Talley, N. J. The mucosal immune system: master regulator of bidirectional gut-brain communications. Nat. Rev. Gastroenterol. Hepatol. 14, 143–159 (2017).
CAS PubMed Article Google Scholar
- 196.
Holtmann, G., Shah, A. & Morrison, M. Pathophysiology of functional gastrointestinal disorders: a holistic overview. Dig. Dis. 35, 5–13 (2017).
PubMed Article Google Scholar
- 197.
Kaerts, N., Van Hal, G., Vermandel, A. & Wyndaele, J. J. Readiness signs used to define the proper moment to start toilet training: a review of the literature. Neurourol. Urodyn. 31, 437–440 (2012).
PubMed Article Google Scholar
- 198.
Michel, R. S. Toilet training. Pediatr. Rev. 20, 240–245 (1999).
CAS PubMed Article Google Scholar
- 199.
Mugie, S. M., Di Lorenzo, C. & Benninga, M. A. Constipation in childhood. Nat. Rev. Gastroenterol. Hepatol. 8, 502–511 (2011).
PubMed Article Google Scholar
- 200.
Solzi, G. & Di Lorenzo, C. Are constipated children different from constipated adults. Dig. Dis. 17, 308–315 (1999).
CAS PubMed Article Google Scholar
- 201.
Di Lorenzo, C. & Benninga, M. A. Pathophysiology of pediatric fecal incontinence. Gastroenterology 126, S33–S40 (2004).
PubMed Article Google Scholar
- 202.
Skardoon, G. R., Khera, A. J., Emmanuel, A. V. & Burgell, R. E. Review article: dyssynergic defaecation and biofeedback therapy in the pathophysiology and management of functional constipation. Aliment. Pharmacol. Ther. 46, 410–423 (2017).
CAS PubMed Article Google Scholar
- 203.
Bharucha, A. & Lacy, B. E. Mechanisms, evaluation, and management of chronic constipation. Gastroenterology 158, 1232–1249 (2020).
CAS PubMed Article Google Scholar
- 204.
Narayanan, S. & Bharucha, A. E. A practical guide to biofeedback therapy for pelvic floor disorders. Curr. Gastroenterol. Rep. 21, 21 (2019).
PubMed Article Google Scholar
- 205.
Rao, S. S., Kavlock, R. & Rao, S. Influence of body position and stool characteristics on defecation in humans. Am. J. Gastroenterol. 101, 2790–2796 (2006).
PubMed Article Google Scholar
- 206.
Sikirov, D. Comparison of straining during defecation in three positions: results and implications for human health. Dig. Dis. Sci. 48, 1201–1205 (2003).
PubMed Article Google Scholar
- 207.
Takano, S., Nakashima, M., Tsuchino, M., Nakao, Y. & Watanabe, A. Influence of foot stool on defecation: a prospective study. Pelviperineology 37, 101–103 (2018).
Google Scholar
- 208.
Rose, C., Parker, A., Jefferson, B. & Cartmell, E. The characterization of feces and urine: a review of the literature to inform advanced treatment technology. Crit. Rev. Env. Sci. Technol. 45, 1827–1879 (2015).
CAS Article Google Scholar
- 209.
Stephen, A. M. & Cummings, J. H. The microbial contribution to human faecal mass. J. Med. Microbiol. 13, 45–56 (1980).
CAS PubMed Article Google Scholar
- 210.
Wierdsma, N. et al. Malabsorption and nutritional balance in the ICU: fecal weight as a biomarker: a prospective observational pilot study. Crit. Care 15, R264 (2011).
PubMed PubMed Central Article Google Scholar
- 211.
Watten, R. H., Morgan, F. M., Yachai Na, S., Vanikiati, B. & Phillips, R. A. Water and electrolyte studies in cholera. J. Clin. Invest. 38, 1879–1889 (1959).
CAS PubMed PubMed Central Article Google Scholar
- 212.
Lewis, S. & Heaton, K. W. Stool form scale as a useful guide to intestinal transit time. Scand. J. Gastroenterol. 32, 920–924 (1997).
CAS PubMed Article Google Scholar
- 213.
Blake, M. R., Raker, J. M. & Whelan, K. Validity and reliability of the Bristol Stool Form Scale in healthy adults and patients with diarrhoea-predominant irritable bowel syndrome. Aliment. Pharmacol. Ther. 44, 693–703 (2016).
CAS PubMed Article Google Scholar
- 214.
Degen, L. P. & Phillips, S. F. How well does stool form reflect colonic transit? Gut 39, 109–113 (1996).
CAS PubMed PubMed Central Article Google Scholar
- 215.
Jarunvongvanich, V., Patcharatrakul, T. & Gonlachanvit, S. Prediction of delayed colonic transit using Bristol stool form and stool frequency in Eastern constipated patients: a difference from the West. J. Neurogastroenterol. Motil. 23, 561–568 (2017).
Article Google Scholar
- 216.
Saad, R. et al. Do stool form and frequency correlate with whole-gut and colonic transit? Results from a multicenter study in constipated individuals and healthy controls. Am. J. Gastroenterol. 105, 403–411 (2010).
PubMed Article Google Scholar
- 217.
Tunc, V. T., Camurdan, A. D., Ilhan, M. N., Sahin, F. & Beyazova, U. Factors associated with defecation patterns in 0–24-month-old children. Eur. J. Pediatr. 167, 1357–1362 (2008).
PubMed Article Google Scholar
- 218.
Bekkali, N., Hamers, S. L., Reitsma, J. B., Van Toledo, L. & Benninga, M. A. Infant stool form scale: development and results. J. Pediatr. 154, 521–526.e1 (2009).
PubMed Article Google Scholar
- 219.
Russo, M. et al. Stool consistency, but not frequency, correlates with total gastrointestinal transit time in children. J. Pediatr. 162, 1188–1192 (2013).
PubMed Article Google Scholar
- 220.
Roager, H. et al. Colonic transit time is related to bacterial metabolism and mucosal turnover in the gut. Nat. Microbiol. 1, 16093 (2016).
CAS PubMed Article Google Scholar
- 221.
Vandeputte, D. et al. Stool consistency is strongly associated with gut microbiota richness and composition, enterotypes and bacterial growth rates. Gut 65, 57–62 (2016).
CAS PubMed Article Google Scholar
- 222.
Kashyap, P. et al. Complex interactions among diet, gastrointestinal transit, and gut microbiota in humanized mice. Gastoenterology 144, 967–977 (2013).
Article Google Scholar
- 223.
Simren, M. et al. Intestinal microbiota in functional bowel disorders: a Rome Foundation report. Gut 62, 159–176 (2013).
PubMed Article Google Scholar
- 224.
Lewis, S. & Heaton, K. W. Increasing butyrate concentration in the distal colon by accelerating intestinal transit. Gut 41, 245–251 (1997).
CAS PubMed PubMed Central Article Google Scholar
- 225.
Tottey, W. et al. Colonic transit time is a driven force of the gut microbiota composition and metabolism: in vitro evidence. J. Neurogastroenterol. Motil. 23, 124–134 (2017).
PubMed PubMed Central Article Google Scholar
- 226.
Wolf, P. et al. Assessing the colonic microbiome, hydrogenogenic and hydrogenotrophic genes, transit and breath methane in constipation. Neurogastroenterol. Motil. 29, 1–9 (2017).
CAS PubMed PubMed Central Article Google Scholar
- 227.
Lee, K., Paik, C. N., Chung, W. C., Yang, J. M. & Choi, M. G. Breath methane positivity is more common and higher in patients with objectively proven delayed transit constipation. Eur. J. Gastroenterol. Hepatol. 25, 726–732 (2013).
PubMed Article Google Scholar
- 228.
Parthasarathy, G. et al. Relationship between microbiota of the colonic mucosa vs feces and symptoms, colonic transit, and methane production in female patients with chronic constipation. Gastoenterology 150, 367–379 (2016).
Article Google Scholar
- 229.
Pittayanon, R. et al. Gut microbiota in patients with irritable bowel syndrome — a systematic review. Gastroenterology 157, 97–108 (2019).
PubMed Article Google Scholar
- 230.
Ghouri, Y. et al. Systematic review of randomized controlled trials of probiotics, prebiotics, and synbiotics in inflammatory bowel disease. Clin. Exp. Gastroenterol. 7, 473–587 (2014).
PubMed PubMed Central Google Scholar
- 231.
Singh, S., Stroud, A. M., Holubar, S. D., Sandborn, W. J. & Pardi, D. S. Treatment and prevention of pouchitis after ileal pouch-anal anastomosis for chronic ulcerative colitis. Cochrane Database Syst. Rev. 11, CD001176 (2015).
Google Scholar
- 232.
Ford, A. et al. Efficacy of prebiotics, probiotics, and synbiotics in irritable bowel syndrome and chronic idiopathic constipation: systematic review and meta-analysis. Am. J. Gastroenterol. 109, 1547–1561 (2014).
PubMed Article Google Scholar
- 233.
Menees, S., Maneerattannaporn, M., Kim, H. M. & Chey, W. D. The efficacy and safety of rifamixin for the irritable bowel syndrome: a systematic review and meta-analysis. Am. J. Gastroenterol. 107, 28–35 (2012).
CAS PubMed Article Google Scholar
- 234.
Lembo, A. et al. Repeat treatment with rifamixin is safe and effective in patients with diarrhea-predominant irritable bowel syndrome. Gastroenterology 151, 1113–1121 (2016).
CAS PubMed Article Google Scholar
- 235.
Dimidi, E., Christodoulides, S., Fragkos, K. C., Scott, S. M. & Whelan, K. The effect of probiotics on functional constipation in adults: a systematic review and meta-analysis of randomized controlled trials. Am. J. Clin. Nutr. 100, 1075–1084 (2014).
CAS PubMed Article Google Scholar
- 236.
Christodoulides, S. et al. Systematic review with meta-analysis: effect of fibre supplementation on chronic idiopathic constipation in adults. Aliment. Pharmacol. Ther. 44, 103–116 (2016).
CAS PubMed Article Google Scholar
- 237.
Allen, S., Martinez, E. G., Gregorio, G. V. & Dans, L. F. Probiotics for treating acute infectious diarrhea. Cochrane Database Syst. Rev. 11, CD003048 (2010).
Google Scholar
- 238.
McFarland, L. Meta-analysis for probiotics for the prevention of traveler's diarrhea. Travel. Med. Infect. Dis. 5, 97–105 (2007).
PubMed Article Google Scholar
- 239.
Bassotti, G., Bucaneve, G., Betti, C. & Morelli, A. Sudden awakening from sleep: effects on proximal and distal colonic contractile activity in man. Eur. J. Gastroenterol. Hepatol. 2, 475–478 (1990).
Google Scholar
- 240.
Furukawa, Y. et al. Relationship between sleep patterns and human colonic motor patterns. Gastroenterology 107, 1372–1381 (1994).
CAS PubMed Article Google Scholar
- 241.
Narducci, F., Bassotti, G., Gaburri, M. & Morelli, A. Twenty four hour manometric recording of colonic motor activity in healthy man. Gut 28, 17–25 (1987).
CAS PubMed PubMed Central Article Google Scholar
- 242.
Barclay, A. Note on the movements of the large intestine. Arch. Roentgen Ray 16, 422–424 (1912).
Article Google Scholar
- 243.
Hertz, A. & Newton, A. The normal movements of the colon in man. J. Physiol. 47, 57–65 (1913).
CAS PubMed PubMed Central Article Google Scholar
- 244.
Ducrotte, P., Denis, P., Bellagha, K. & Riachi, G. Motor response of the digestive tract to food. Gastroenterol. Clin. Biol. 18, 157–164 (1994).
CAS PubMed Google Scholar
- 245.
Rogers, J., Raimundo, A. H. & Misiewicz, J. J. Cephalic phase of colonic pressure response to food. Gut 34, 537–543 (1993).
CAS PubMed PubMed Central Article Google Scholar
- 246.
Holdstock, D. & Misiewicz, J. J. Factors controlling colonic motility: colonic pressures and transit after meals in patients with total gastrectomy, pernicious anaemia or duodenal ulcer. Gut 11, 100–110 (1970).
CAS PubMed PubMed Central Article Google Scholar
- 247.
Snape, W. J. Jr, Matarazzo, S. A. & Cohen, S. Effect of eating and gastrointestinal hormones on human colonic myoelectrical and motor activity. Gastroenterology 75, 373–378 (1978).
CAS PubMed Article Google Scholar
- 248.
Rao, S. S., Kavelock, R., Beaty, J., Ackerson, K. & Stumbo, P. Effects of fat and carbohydrate meals on colonic motor response. Gut 46, 205–211 (2000).
CAS PubMed PubMed Central Article Google Scholar
- 249.
Di Stefano, M., Miceli, E., Missanelli, A., Mazzocchi, S. & Corazza, G. R. Meal induced rectosigmoid tone modification: a low caloric meal accurately separates functional and organic gastrointestinal disease patients. Gut 55, 1409–1414 (2006).
PubMed PubMed Central Article Google Scholar
- 250.
Aaronson, M. J., Freed, M. M. & Burakoff, R. Colonic myoelectric activity in persons with spinal cord injury. Dig. Dis. Sci. 30, 295–300 (1985).
CAS PubMed Article Google Scholar
- 251.
Snape, W. J. Jr, Wright, S. H., Battle, W. M. & Cohen, S. The gastrocolic response: evidence for a neural mechanism. Gastroenterology 77, 1235–1240 (1979).
PubMed Article Google Scholar
- 252.
Bassotti, G., Clementi, M., Antonelli, E., Pelli, M. A. & Tonini, M. Low-amplitude propagated contractile waves: a relevant propulsive mechanism of human colon. Dig. Liver Dis. 33, 36–40 (2001).
CAS PubMed Article Google Scholar
- 253.
Herbst, F. et al. Gastrointestinal transit and prolonged ambulatory colonic motility in health and faecal incontinence. Gut 41, 381–389 (1997).
CAS PubMed PubMed Central Article Google Scholar
- 254.
Dominianni, C. et al. Sex, body mass index, and dietary fiber intake influence the human gut microbiome. PLoS ONE 10, e0124599 (2015).
PubMed PubMed Central Article CAS Google Scholar
- 255.
Stephen, A. & Cummings, J. H. Mechanism of action of dietary fibre in the human colon. Nature 284, 283–284 (1980).
CAS PubMed Article Google Scholar
- 256.
Gill, S., Rossi, M., Bajka, B. & Whelan, K. Dietary fibre in gastrointestinal health and disease. Nat. Rev. Gastroenterol. Hepatol. 18, 101–116 (2020).
PubMed Article CAS Google Scholar
- 257.
Stephen, A., Wiggins, H. S. & Cummings, J. S. Effect of changing transit time on colonic microbial metabolism in man. Gut 28, 601–609 (1987).
CAS PubMed PubMed Central Article Google Scholar
- 258.
Gibson, G. et al. The International Scientific Association for Probiotics and Prebiotics (ISAPP) consensus statement on the definition and scope of prebiotics. Nat. Rev. Gastroenterol. Hepatol. 14, 491–500 (2019).
Article Google Scholar
- 259.
Shen, D. et al. Positive effects of resistant starch supplementation on bowel function in healthy adults: a systematic review and meta-analysis of randomized controlled trials. Int. J. Food Sci. Nutr. 68, 149–157 (2017).
CAS PubMed Article Google Scholar
- 260.
Wilkinson-Smith, V. et al. Mechanisms underlying effects of kiwifruit on intestinal function shown by MRI in healthy volunteers. Aliment. Pharmacol. Ther. 49, 759–768 (2019).
CAS PubMed PubMed Central Article Google Scholar
- 261.
Murray, K. et al. Differential effects of FODMAPs (fermentable oligo-, di-, mono-saccharides and polyols) on small and large intestinal contents in healthy subjects shown by MRI. Am. J. Gastroenterol. 109, 110–119 (2013).
PubMed PubMed Central Article CAS Google Scholar
- 262.
de Vries, J., Birkett, A., Hulshof, T., Verbeke, K. & Gibes, K. Effects of cereal, fruit and vegetable fibers on human fecal weight and transit time: a comprehensive review of intervention trials. Nutrients 8, 130 (2016).
PubMed PubMed Central Article CAS Google Scholar
- 263.
Cummings, J. in CRC Handbook on Dietary Fiber in Nutrition (ed. Spiller, G. S.) 263–349 (CRC Press, 1993).
- 264.
de Vries, J., Miller, P. E. & Verbeke, K. Effects of cereal fiber on bowel function: a systematic review of intervention trials. World J. Gastroenterol. 21, 8952–8963 (2015).
PubMed PubMed Central Article Google Scholar
- 265.
Talley, N., Jones, M., Nuyts, G. & Dubois, D. Risk factors for chronic constipation based on a general practice sample. Am. J. Gastroenterol. 98, 1107–1111 (2003).
PubMed Article Google Scholar
- 266.
Chiarelli, P., Brown, W. & McElduff, P. Constipation in Australian women: prevalence and associated factors. Int. Urogynecol. J. Pelvic Floor. Dysfunct. 11, 71–78 (2000).
CAS PubMed Article Google Scholar
- 267.
Suares, N. & Ford, A. C. Prevalence of, and risk factors for, chronic idiopathic constipation in the community: systematic review and meta-analysis. Am. J. Gastroenterol. 106, 1582–1591 (2011).
PubMed Article Google Scholar
- 268.
Johanson, J. & Sonnenberg, A. The prevalence of hemorrhoids and chronic constipation an epidemiologic study. Gastroenterology 98, 360–363 (1990).
Article Google Scholar
- 269.
Sandler, R., Jordan, M. C. & Shelton, B. J. Demographic and dietary determinants of constipation in the US population. Am. J. Public Health 80, 185–189 (1990).
CAS PubMed PubMed Central Article Google Scholar
- 270.
Palsson, O., Whitehead, W., Törnblom, H., Sperber, A. D. & Simren, M. Prevalence of Rome IV functional bowel disorders among adults in the United States, Canada, and the United Kingdom. Gastroenterology 158, 1262–1273 (2020).
PubMed Article Google Scholar
- 271.
Madsen, J. L. & Graff, J. Effects of ageing on gastrointestinal motor function. Age Ageing 33, 154–159 (2004).
PubMed Article Google Scholar
- 272.
Graff, J., Brinch, K. & Madsen, J. L. Gastrointestinal mean transit times in young and middle-aged healthy subjects. Clin. Physiol. 21, 253–259 (2001).
CAS PubMed Article Google Scholar
- 273.
Broad, J. et al. Changes in neuromuscular structure and functions of human colon during ageing are region-dependent. Gut 68, 1210–1223 (2019).
CAS PubMed Article Google Scholar
- 274.
Kim, S. Colonic slow transit can cause changes in the gut environment observed in the elderly. J. Neurogastroenterol. Motil. 23, 3–4 (2017).
PubMed PubMed Central Article Google Scholar
- 275.
Mugie, S., Benninga, M. A. & Di Lorenzo, C. Epidemiology of constipation in children and adults: a systematic review. Best Pract. Res. Clin. Gastroenterol. 25, 3–18 (2011).
PubMed Article Google Scholar
- 276.
Metcalf, A. et al. Simplified assessment of segmental colonic transit. Gastroenterology 92, 40–47 (1987).
CAS PubMed Article Google Scholar
- 277.
Sadik, R., Abrahamsson, H. & Stotzer, P. O. Gender differences in gut transit shown with a newly developed radiological procedure. Scand. J. Gastroenterol. 38, 36–42 (2003).
CAS PubMed Article Google Scholar
- 278.
Abrahamsson, H., Antov, S. & Bosaeus, I. Gastrointestinal and colonic segmental transit time evaluated by a single abdominal X-ray in healthy subjects and constipated patients. Scand. J. Gastroenterol. Suppl. 152, 72–80 (1988).
CAS PubMed Article Google Scholar
- 279.
Meier, R. et al. Influence of age, gender, hormonal status and smoking habits on colonic transit time. Neurogastroenterol. Motil. 7, 235–238 (1995).
CAS PubMed Article Google Scholar
- 280.
Degen, L. P. & Phillips, S. F. Variability of gastrointestinal transit in healthy women and men. Gut 39, 299–305 (1996).
CAS PubMed PubMed Central Article Google Scholar
- 281.
Zutshi, M., Hull, T. L., Bast, J. & Hammel, J. Female bowel function: the real story. Dis. Colon. Rectum 50, 351–358 (2007).
CAS PubMed Article Google Scholar
- 282.
Heitkemper, M. & Chang, L. Do fluctuations in ovarian hormones affect gastrointestinal symptoms in women with irritable bowel syndrome? Gend. Med. 6, 152–167 (2009).
PubMed PubMed Central Article Google Scholar
- 283.
Gonenne, J. et al. Effect of female sex hormone supplementation and withdrawal on gastrointestinal and colonic transit in postmenopausal women. Neurogastroenterol. Motil. 18, 911–918 (2006).
CAS PubMed Article Google Scholar
- 284.
Wald, A. et al. Gastrointestinal transit: the effect of the menstrual cycle. Gastroenterology 80, 1497–1500 (1981).
CAS PubMed Article Google Scholar
- 285.
Jung, H. K., Kim, D. Y. & Moon, I. H. Effects of gender and menstrual cycle on colonic transit time in healthy subjects. Korean J. Intern. Med. 18, 181–186 (2003).
PubMed PubMed Central Article Google Scholar
- 286.
Townsend, D. et al. Pathophysiology of fecal incontinence differs between men and women: a case-matched study in 200 patients. Neurogastroenterol. Motil. 28, 1580–1588 (2016).
CAS PubMed Article Google Scholar
- 287.
Jameson, J. et al. Effect of age, sex and parity on anorectal function. Br. J. Surg. 81, 1689–1692 (1994).
CAS PubMed Article Google Scholar
- 288.
Abramowitz, L. et al. Are sphincter defects the cause of anal incontinence after vaginal delivery? Dis. Colon. Rectum 43, 590–596 (2000).
CAS PubMed Article Google Scholar
- 289.
Rieger, N., Schloithe, A. C., Saccone, G. & Wattchow, D. A prospective study of anal sphincter injury due to childbirth. Scand. J. Gastroenterol. 33, 950–955 (1998).
CAS PubMed Article Google Scholar
- 290.
Bharucha, A. E., Fletcher, J. G., Melton, L. J. 3rd & Zinsmeister, A. R. Obstetric trauma, pelvic floor injury and fecal incontinence: a population-based case-control study. Am. J. Gastroenterol. 107, 902–911 (2012).
PubMed PubMed Central Article Google Scholar
- 291.
Oberwalder, M., Connor, J. & Wexner, S. D. Meta-analysis to determine the incidence of obstetric anal sphincter damage. Br. J. Surg. 90, 1333–1337 (2003).
CAS PubMed Article Google Scholar
- 292.
Bharucha, A. E. et al. Prevalence and burden of fecal incontinence: a population-based study in women. Gastroenterology 129, 42–49 (2005).
PubMed Article Google Scholar
- 293.
Larsson, C. et al. Anal incontinence after caesarean and vaginal delivery in Sweden: a national population-based study. Lancet 393, 1233–1239 (2019).
PubMed Article Google Scholar
- 294.
Lunniss, P. J., Gladman, M. A., Hetzer, F. H., Williams, N. S. & Scott, S. M. Risk factors in acquired faecal incontinence. J. R. Soc. Med. 97, 111–116 (2004).
PubMed PubMed Central Article Google Scholar
- 295.
Boyle, D. J. et al. The effects of age and childbirth on anal sphincter function and morphology in 999 symptomatic female patients with colorectal dysfunction. Dis. Colon. Rectum 55, 286–293 (2012).
CAS PubMed Article Google Scholar
- 296.
Heitmann, P. T. et al. Relationships between the results of anorectal investigations and symptom severity in patients with faecal incontinence. Int. J. Colorectal Dis. 34, 1445–1454 (2019).
CAS PubMed Article Google Scholar
- 297.
Heitmann, P. et al. The relationships between the results of contemporary tests of anorectal structure and sensorimotor function and the severity of faecal incontinence. Neurogastroenterol. Motil. 32, e13946 (2020).
PubMed Google Scholar
- 298.
Delgado-Aros, S., Camilleri, M., Garcia, M. A., Burton, D. & Busciglio, I. High body mass alters colonic sensory-motor function and transit in humans. Am. J. Physiol. Gastrointestinal. Liver Physiol. 295, G382–G388 (2008).
CAS Article Google Scholar
- 299.
Aro, P. et al. Body mass index and chronic unexplained gastrointestinal symptoms: an adult endoscopic population based study. Gut 54, 1377–1383 (2005).
CAS PubMed PubMed Central Article Google Scholar
- 300.
Bharucha, A. E., Zinsmeister, A. R., Schleck, C. D. & Melton, L. J. Bowel disturbances are the most important risk factors for late onset fecal incontinence: a population-based case-control study in women. Gastroenterology 139, 1559–1566 (2010).
PubMed Article Google Scholar
- 301.
Manchikanti, L. et al. Opioid epidemic in the United States. Pain Physician 15, ES9–ES38 (2012).
PubMed Article Google Scholar
- 302.
Kalso, E., Edwards, J. E., Moore, R. A. & McQuay, H. J. Opioids in chronic non-cancer pain: systematic review of efficacy and safety. Pain 112, 372–380 (2004).
CAS PubMed Article Google Scholar
- 303.
Bell, T. J. et al. The prevalence, severity, and impact of opioid-induced bowel dysfunction: results of a US and European Patient Survey (PROBE 1). Pain Med. 10, 35–42 (2009).
PubMed Article Google Scholar
- 304.
Vollebregt, P. et al. Association between opioid usage and rectal dysfunction in constipation: a cross-sectional study of 2754 patients. Neurogastroenterol. Motil. 32, e13839 (2020).
CAS PubMed Article Google Scholar
- 305.
Iovino, P. et al. New onset of constipation during long-term physical inactivity: a proof-of-concept study on the immobility-induced bowel changes. PLoS ONE 8, e72608 (2013).
CAS PubMed PubMed Central Article Google Scholar
- 306.
Strid, H., Simrén, M., Störsrud, S., Stotzer, P. O. & Sadik, R. Effect of heavy exercise on gastrointestinal transit in endurance athletes. Scand. J. Gastroenterol. 46, 673–677 (2011).
PubMed Article Google Scholar
- 307.
Lacy, B. et al. Bowel disorders. Gastoenterology 150, 1393–1407 (2016).
Article Google Scholar
- 308.
Rao, S. S. et al. Functional anorectal disorders. Gastroenterology 130, 1510–1518 (2016).
Google Scholar
- 309.
Rey, E., Balboa, A. & Mearin, F. Chronic constipation, irritable bowel syndrome with constipation and constipation with pain/discomfort: similarities and differences. Am. J. Gastroenterol. 109, 876–884 (2014).
PubMed Article Google Scholar
- 310.
Shekhar, C. et al. Rome III functional constipation and irritable bowel syndrome with constipation are similar disorders within a spectrum of sensitization, regulated by serotonin. Gastroenterology 145, 749–757 (2013).
CAS PubMed Article Google Scholar
- 311.
Wong, R. et al. Inability of the Rome III criteria to distinguish functional constipation from constipation-subtype irritable bowel syndrome. Am. J. Gastroenterol. 105, 2228–2234 (2010).
PubMed PubMed Central Article Google Scholar
- 312.
Whitehead, W., Palsson, O. S. & Simren, M. Biomarkers to distinguish functional constipation from irritable bowel syndrome with constipation. Neurogastroenterol. Motil. 28, 783–792 (2016).
CAS PubMed Article Google Scholar
- 313.
Bharucha, A. et al. Differences between painless and painful constipation among community women. Am. J. Gastroenterol. 101, 604–612 (2006).
PubMed Article Google Scholar
- 314.
Ford, A. et al. Characteristics of functional bowel disorder patients: a cross-sectional survey using the Rome III criteria. Aliment. Pharmacol. Ther. 39, 312–321 (2014).
CAS PubMed Article Google Scholar
- 315.
Palsson, O., Baggish, J. S., Turner, M. J. & Whitehead, W. E. IBS patients show frequent fluctuations between loose/watery and hard/lumpy stools: implications for treatment. Am. J. Gastroenterol. 107, 286–295 (2012).
PubMed Article Google Scholar
- 316.
Dinning, P., Carrington, E. V. & Scott, S. M. The use of colonic and anorectal high-resolution manometry and its place in clinical work and in research. Neurogastroenterol. Motil. 27, 1693–1708 (2015).
CAS PubMed Article Google Scholar
- 317.
Brochard, C. et al. Quality of life in 1870 patients with constipation and/or fecal incontinence: constipation should not be underestimated. Clin. Res. Hepatol. Gastroenterol. 43, 682–687 (2019).
PubMed Article Google Scholar
- 318.
Cohen, M. et al. Evaluation of interstitial cells of Cajal in patients with severe colonic inertia requiring surgery: a clinical-pathological study. Colorectal Dis. 19, 462–466 (2017).
CAS PubMed Article Google Scholar
- 319.
Lee, J., Park, H., Kamm, M. A. & Talbot, I. C. Decreased density of interstitial cells of Cajal and neuronal cells in patients with slow-transit constipation and acquired megacolon. J. Gastroenterol. Hepatol. 20, 1292–1298 (2005).
PubMed Article Google Scholar
- 320.
Dinning, P. G. et al. Pancolonic spatiotemporal mapping reveals regional deficiencies in, and disorganization of colonic propagating pressure waves in severe constipation. Neurogastroenterol. Motil. 22, e340–e349 (2010).
CAS PubMed Article Google Scholar
- 321.
Dinning, P. et al. High-resolution colonic motility recordings in vivo compared with ex vivo recordings after colectomy, in patients with slow transit constipation. Neurogastroenterol. Motil. 28, 1824–1835 (2016).
CAS PubMed Article Google Scholar
- 322.
Camilleri, M. et al. Chronic constipation. Nat. Rev. Dis. Primers 3, 17095 (2017).
PubMed Article Google Scholar
- 323.
Bassotti, G. et al. Colonic neuropathological aspects in patients with intractable constipation due to obstructed defecation. Mod. Pathol. 20, 367–374 (2007).
PubMed Article Google Scholar
- 324.
Bassotti, G. & Villanacci, V. Can "functional" constipation be considered as a form of enteric neuro-gliopathy? Glia 59, 345–350 (2010).
PubMed Article Google Scholar
- 325.
Bassotti, G., Villanacci, V., Creţoiu, D., Creţoiu, S. M. & Becheanu, G. Cellular and molecular basis of chronic constipation: taking the functional/idiopathic label out. World J. Gastroenterol. 19, 4099–4105 (2013).
PubMed PubMed Central Article CAS Google Scholar
- 326.
Bazzocchi, G. et al. Effect of eating on colonic motility and transit in patients with functional diarrhea. Simultaneous scintigraphic and manometric evaluations. Gastroenterology 101, 1298–1306 (1991).
CAS PubMed Article Google Scholar
- 327.
Tack, J. Functional diarrhea. Gastroenterol. Clin. North Am. 41, 629–637 (2012).
PubMed Article Google Scholar
- 328.
Simrén, M. et al. Visceral hypersensitivity is associated with GI symptom severity in functional GI disorders: consistent findings from five different patient cohorts. Gut 67, 255–262 (2018).
PubMed Article Google Scholar
- 329.
Hong, J. et al. Altered brain responses in subjects with irritable bowel syndrome during cued and uncued pain expectation. Neurogastroenterol. Motil. 28, 127–138 (2016).
PubMed Article Google Scholar
- 330.
Koloski, N., Jones, M. P. & Talley, N. J. Evidence that independent gut-to-brain and brain-to-gut pathways operate in the irritable bowel syndrome and functional dyspepsia: a 1-year population-based prospective study. Aliment. Pharmacol. Ther. 44, 592–600 (2016).
CAS PubMed Article Google Scholar
- 331.
Wang, L. et al. Gut microbial dysbiosis in the irritable bowel syndrome: a systematic review and meta-analysis of case-control studies. J. Acad. Nutr. Dietetics 120, 565–586 (2020).
Article Google Scholar
- 332.
Jalanka, J., Salonen, A., Fuentes, S. & de Vos, W. M. Microbial signatures in post-infectious irritable bowel syndrome — toward patient stratification for improved diagnostics and treatment. Gut Microbes 6, 364–369 (2015).
PubMed PubMed Central Article Google Scholar
- 333.
Shulman, R., Jarrett, M. E., Cain, K. C., Broussard, E. K. & Heitkemper, M. M. Associations among gut permeability, inflammatory markers, and symptoms in patients with irritable bowel syndrome. J. Gastroenterol. 49, 1467–1476 (2014).
CAS PubMed PubMed Central Article Google Scholar
- 334.
Camilleri, M., Madsen, K., Spiller, R., Greenwood-Van Meerveld, B. & Verne, G. N. Intestinal barrier function in health and gastrointestinal disease. Neurogastroenterol. Motil. 24, 503–512 (2012).
CAS PubMed PubMed Central Article Google Scholar
- 335.
Camilleri, M. Peripheral mechanisms in irritable bowel syndrome. N. Engl. J. Med. 367, 1626–1635 (2012).
CAS PubMed Article Google Scholar
- 336.
Ford, A. & Talley, N. J. Mucosal inflammation as a potential etiological factor in irritable bowel syndrome: a systematic review. J. Gastroenterol. Hepatol. 46, 421–431 (2011).
Google Scholar
- 337.
Elli, L. et al. Evidence for the presence of non-celiac gluten sensitivity in patients with functional gastrointestinal symptoms: results from a multicenter randomized double-blind placebo-controlled gluten challenge. Nutrients 8, 84 (2016).
PubMed PubMed Central Article CAS Google Scholar
- 338.
Beyder, A. et al. Loss-of-function of the voltage-gated sodium channel NaV1.5 (channelopathies) in patients with irritable bowel syndrome. Gastroenterology 146, 1659–1668 (2014).
CAS PubMed Article Google Scholar
- 339.
Valentin, N. et al. Biomarkers for bile acid diarrhea in functional bowel disorder with diarrhea: a systematic review and meta-analysis. Gut 65, 1951–1959 (2015).
PubMed Article CAS Google Scholar
- 340.
Shin, A. et al. Bowel functions, fecal unconjugated primary and secondary bile acids, and colonic transit in patients with irritable bowel syndrome. Clin. Gastroenterol. Hepatol. 11, 1270–1275 (2013).
CAS PubMed PubMed Central Article Google Scholar
- 341.
Muñoz-Yagüe, T. et al. Fecal incontinence in men: causes and clinical and manometric features. World J. Gastroenterol. 20, 7933–7940 (2014).
PubMed PubMed Central Article Google Scholar
- 342.
Bharucha, A. E. et al. Relationship between symptoms and disordered continence mechanisms in women with idiopathic faecal incontinence. Gut 54, 546–555 (2005).
CAS PubMed PubMed Central Article Google Scholar
- 343.
Dietz, H. Pelvic organ prolapse — a review. Aust. Fam. Physician 44, 446–452 (2015).
PubMed Google Scholar
- 344.
Kiff, E. S. & Swash, M. Slowed conduction in the pudendal nerves in idiopathic (neurogenic) faecal incontinence. Br. J. Surg. 71, 614–616 (1984).
CAS PubMed Article Google Scholar
- 345.
Kiff, E., Barnes, P. R. & Swash, M. Evidence of pudendal neuropathy in patients with perineal descent and chronic straining at stool. Gut 25, 1279–1282 (1984).
CAS PubMed PubMed Central Article Google Scholar
- 346.
Swash, M., Snooks, S. J. & Henry, M. M. Unifying concept of pelvic floor disorders and incontinence. J. R. Soc. Med. 78, 906–911 (1985).
CAS PubMed PubMed Central Article Google Scholar
- 347.
Gosselink, M. P. & Joshi, H. M. Exploring the link between high grade internal rectal prolapse and faecal incontinence. Colorectal Dis. 19, 711–712 (2017).
PubMed Article Google Scholar
- 348.
Hawkins, A. T. et al. Impact of rising grades of internal rectal intussusception on fecal continence and symptoms of constipation. Dis. Colon. Rectum 59, 54–61 (2016).
PubMed Article Google Scholar
- 349.
Chan, C., Lunniss, P. J., Wang, D., Williams, N. S. & Scott, S. M. Rectal sensorimotor dysfunction in patients with urge faecal incontinence: evidence from prolonged manometric studies. Gut 54, 1263–1272 (2005).
CAS PubMed PubMed Central Article Google Scholar
- 350.
Gladman, M. A., Lunniss, P. J., Scott, S. M. & Swash, M. Rectal hyposensitivity. Am. J. Gastroenterol. 101, 1140–1151 (2006).
PubMed Article Google Scholar
- 351.
Scott, S. & Lunniss, P. J. Rectal hyposensitivity and functional hindgut disorders: cause and effect or an epiphenomenon? J. Pediatr. Gastroenterol. Nutr. 53 (Suppl. 2), 47–49 (2011).
Google Scholar
- 352.
Chan, C., Scott, S. M., Williams, N. S. & Lunniss, P. J. Rectal hypersensitivity worsens stool frequency, urgency, and lifestyle in patients with urge fecal incontinence. Dis. Colon. Rectum 48, 134–140 (2005).
PubMed Article Google Scholar
- 353.
Aitchison, M. et al. Impaired anal sensation and early diabetic faecal incontinence. Diabet. Med. 8, 960–963 (1991).
CAS PubMed Article Google Scholar
- 354.
Mundet, L. et al. Defective conduction of anorectal afferents is a very prevalent pathophysiological factor associated to fecal incontinence in women. J. Neurogastroenterol. Motil. 25, 423–435 (2019).
PubMed PubMed Central Article Google Scholar
- 355.
Bharucha, A. E. et al. Epidemiology, pathophysiology, and classification of fecal incontinence: state of the science summary for the National Institute of Diabetes and Digestive and Kidney Diseases (NIDDK) workshop. Am. J. Gastroenterol. 110, 127–136 (2015).
PubMed Article Google Scholar
- 356.
Morandi, C., Martellucci, J., Talento, P. & Carriero, A. Role of enterocele in the obstructed defecation syndrome (ODS): a new radiological point of view. Colorectal Dis. 12, 810–816 (2010).
CAS PubMed Article Google Scholar
- 357.
Serrano Falcón, B., Barceló López, M., Mateos Muñoz, B., Álvarez Sánchez, A. & Rey, E. Fecal impaction: a systematic review of its medical complications. BMC Geriatr. 16, 4 (2016).
PubMed PubMed Central Article CAS Google Scholar
- 358.
van der Plas, R. et al. Megarectum in constipation. Arch. Dis. Child. 83, 52–58 (2000).
PubMed PubMed Central Article Google Scholar
- 359.
Lunniss, P., Gladman, M. A., Benninga, M. A. & Rao, S. S. Pathophysiology of evacuation disorders. Neurogastroenterol. Motil. 21(Suppl. 2), 31–40 (2009).
PubMed Article Google Scholar
- 360.
D'Hoore, A. & Penninckx, F. Obstructed defecation. Colorect Dis. 5, 280–287 (2003).
Article Google Scholar
- 361.
Rao, S. & Patcharatrakul, T. Diagnosis and treatment of dyssynergic defecation. J. Neurogastroenterol. Motil. 22, 423–435 (2016).
PubMed PubMed Central Article Google Scholar
- 362.
Rostamania, G., Abramowitch, S., Chang, C. & Goldberg, R. P. Descent and hypermobility of the rectum in women with obstructed defecation symptoms. Int. Urogynecol. J. 31, 337–349 (2020).
Article Google Scholar
- 363.
Bharucha, A. et al. Anal sphincteric neurogenic injury in asymptomatic nulliparous women and fecal incontinence. Am. J. Physiol. Gastrointest. Liver Physiol. 303, G256–G262 (2012).
CAS PubMed PubMed Central Article Google Scholar
Download references
Acknowledgements
P.T.H. is the recipient of the Peter King Research Scholarship, Royal Australasian College of Surgeons.
Ethics declarations
Competing interests
The authors declare no competing interests.
Additional information
Peer review information
Nature Reviews Gastroenterology & Hepatology thanks G. Bassotti, M. Camilleri and J. Keller for their contribution to the peer review of this work.
Publisher's note
Springer Nature remains neutral with regard to jurisdictional claims in published maps and institutional affiliations.
Supplementary information
Rights and permissions
About this article
Cite this article
Heitmann, P.T., Vollebregt, P.F., Knowles, C.H. et al. Understanding the physiology of human defaecation and disorders of continence and evacuation. Nat Rev Gastroenterol Hepatol 18, 751–769 (2021). https://doi.org/10.1038/s41575-021-00487-5
Download citation
-
Accepted:
-
Published:
-
Issue Date:
-
DOI : https://doi.org/10.1038/s41575-021-00487-5
Further reading
-
Break the taboo with poo
Nature Reviews Gastroenterology & Hepatology (2021)
Anal Fistula Chapter 42 Lower Gastrointestinal Problems-10th Edition-lewis
Source: https://www.nature.com/articles/s41575-021-00487-5?proof=t